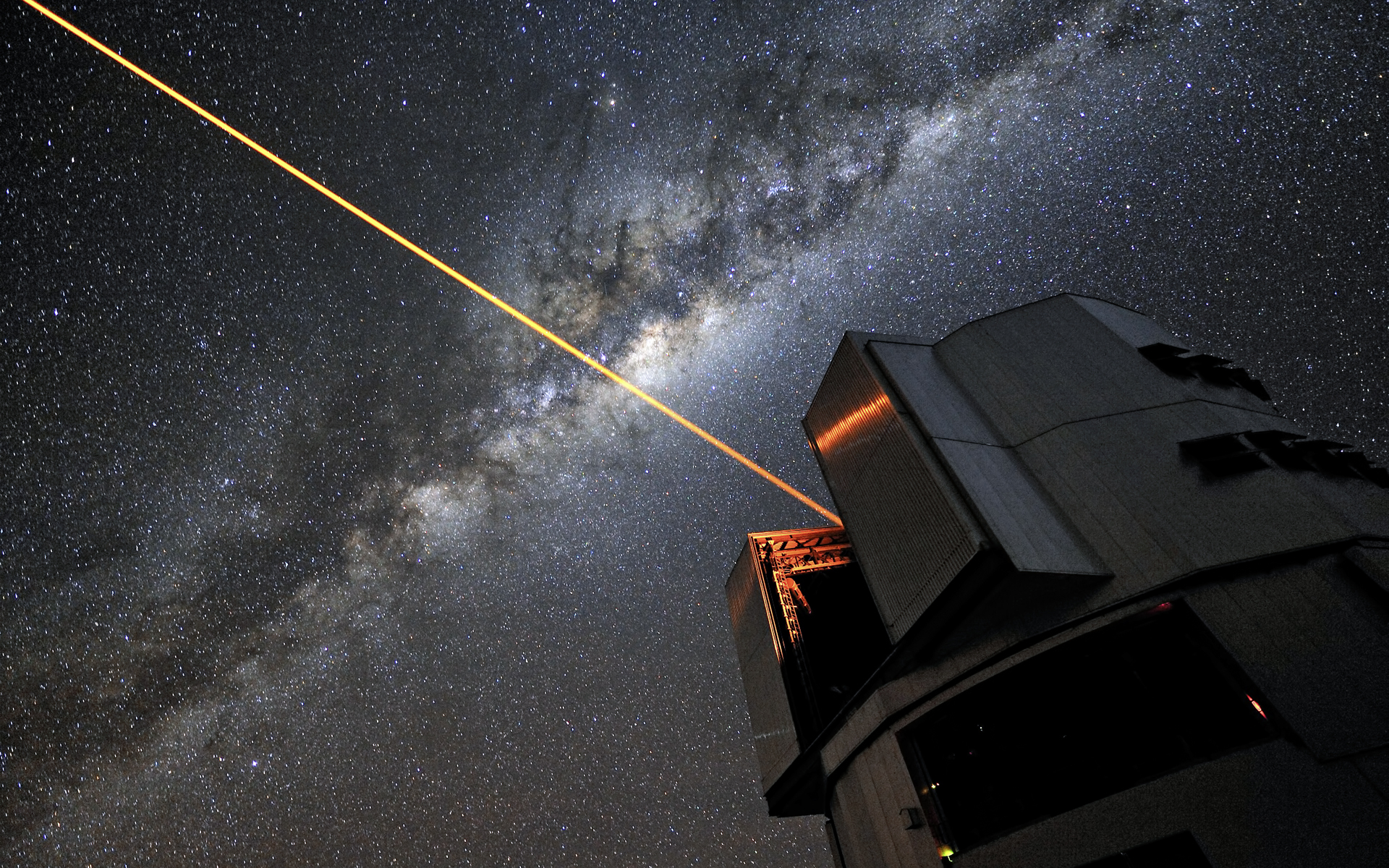
The Very Large Telescope (VLT) uses multiple systems of adaptive optics including deformable mirror system to take the clearest possible images of space. Courtesy of The Daily Galaxy.
Adaptive Optics Introduction
The field of optics studies the behavior of light – a form of substance with a characteristic wavelengths ranging from about 400 to 700 nanometers for visible light to as small as 10 nanometers for the UV end of the spectrum. To gain a perspective on how small those numbers are, consider this: a sheet of paper is 100,000 nanometers thick. This means that in applications and studies of light, precision is vital. Moreover, light waves contain wavefronts – theoretical surfaces that represent the points on a wave in which the wave is propagating in phase.
Wavefront measurements play an important role in various imaging systems that rely on the focusing properties of optical elements. For simplicity it’s enough to consider that preserving the uniformity of the wavefront as light propagates and gets recorded is a cornerstone ingredient of a precise imaging system. Complicating this is the fact that these imaging systems are very sensitive to external changes of ambient conditions, which cannot necessarily be easily controlled. Air currents, temperature or variance in medium density can create variations that are large enough to induce material disturbances in imaging systems. Let’s recall here the nm-scales of precision that these instruments mandate.
Wavefront distortions cause an incoming wave to focus at different points in space. Researchers are now able to correct image imperfections caused by wavefront distortion by installing adaptive optics systems. The most common device used for correcting distortions of waveform is the deformable mirror.
What is a Deformable Mirror?
Deformable mirrors have a surface that can be misshapen to control wavefronts. The mirror changes shape in real-time to respond to wavefront distortion and eliminate resulting image imperfections. Removing optical imperfections (known as aberrations) provides the highest resolution image possible. Researchers use deformable mirrors in combination with wavefront sensors and control systems to correct incoming distorted wavefronts. The mirrors are formed on actuators- small mechanical components that allow the mirror to tilt and tip very quickly. This allows the device to have many degrees of freedom, therefore providing the smoothest possible wavefront control.
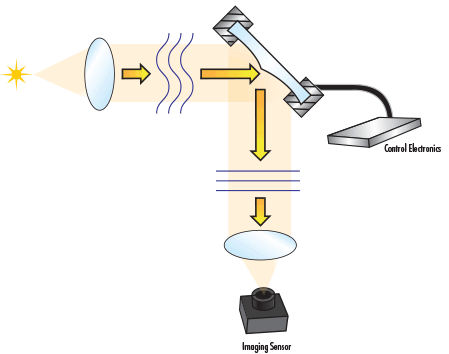
A misshapen wave hits the controlled deformable mirror, and the corrected wave is viewed by the imaging system. Courtesy of Edmund Optics.
Parameters of a Deformable Mirror
Researchers are able to choose the deformable mirror that best suits their application based on certain features. These parameters include the following:
- Number of Actuators: This number determines the versatility in deformation and typically ranges from tens to hundreds.
- Dimensions: Deformable mirrors range in size from a few millimeters to full meters because deformable mirrors are used in both micro and macro applications.
- Surface Type: Deformable mirrors are continuous or segmented. Continuous mirrors have a single surface deformable at various points. Segmented mirrors have many individually controlled small mirror sections.
- Response Time: Response time determines how quickly the mirror responds to the control signal. This typically varies from microseconds to tens of seconds.
- Actuator Stroke: This distance is the maximum distance each individual actuator can move from its central position. Strokes typically range from 1 to 30 micrometers in the positive and negative direction.
Applications of Deformable Mirrors
Deformable mirrors play a crucial role in enhancing the performance of contemporary imaging systems by correcting wavefront aberrations. These sophisticated optical elements are utilized across a broad spectrum of applications, ranging from astronomical observations to medical imaging. In the vast expanse of space, deformable mirrors are integral to telescopes, enabling astronomers to capture clear, high-resolution images of distant celestial bodies by compensating for the distortion caused by the Earth’s atmosphere. This technology allows for the observation of intricate details on the surfaces of stars and planets that would otherwise be obscured by atmospheric turbulence.
On a much smaller scale, deformable mirrors are also employed in the field of ophthalmology to improve the clarity of images of the human retina. This application is particularly significant for diagnosing and treating retinal diseases, where the ability to precisely visualize minute retinal structures can be the key to preventing vision loss. By dynamically adjusting their shape in response to real-time feedback, deformable mirrors can correct for aberrations caused by irregularities in the eye’s optical properties, resulting in sharper and more detailed images.
The effectiveness of deformable mirrors is greatly enhanced by their integration with advanced real-time computing systems. These systems analyze the incoming wavefronts and calculate the necessary adjustments in microseconds, enabling the mirrors to adapt to rapidly changing conditions and correct even severe distortions. This synergy between adaptive optics and high-speed computing leads to unprecedented levels of image resolution and clarity, pushing the boundaries of what can be observed and analyzed in both astronomical and biomedical contexts.
Retinal Imaging
Retinal imaging systems take pictures of the back of the human eye to detect for certain diseases and determine overall health of the eye. Light coming out from the eye often carries ocular aberrations as it exits the pupil, thereby making it difficult to image the microscopic structure of the retina. High-order aberrations such as coma and spherical aberration are subject to change over short periods of time and therefore require continuous measurement and correction. These aberrations are typically caused by a misshapen lens and can be measured by a wavefront sensor (most often a Shack-Hartmann). The sensor then communicates with a system of deformable mirrors for correction. This results in a clear and detailed account of the retina for inspection.
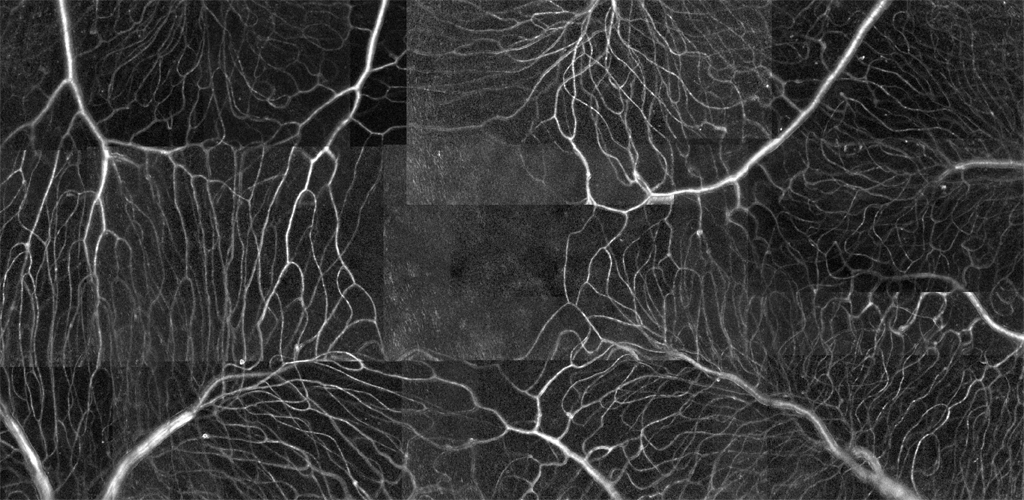
Doctors can use deformable mirrors in advanced retinal imaging systems to view signs of disease or health problems. Courtesy of University of Rochester
Astronomical Imaging
Adaptive optics and deformable mirrors are perhaps the most crucial advancements in space imaging. Incoming light from space endures much turbulence when it hits the Earth’s atmosphere, therefore causing major distortions in wavefronts and thus producing severely blurred images. With deformable mirrors, ground-based telescopes are now able to obtain images almost as sharp as those observed by space telescopes. These corrections allow astronomers to view detailed images of much fainter objects than is otherwise possible.
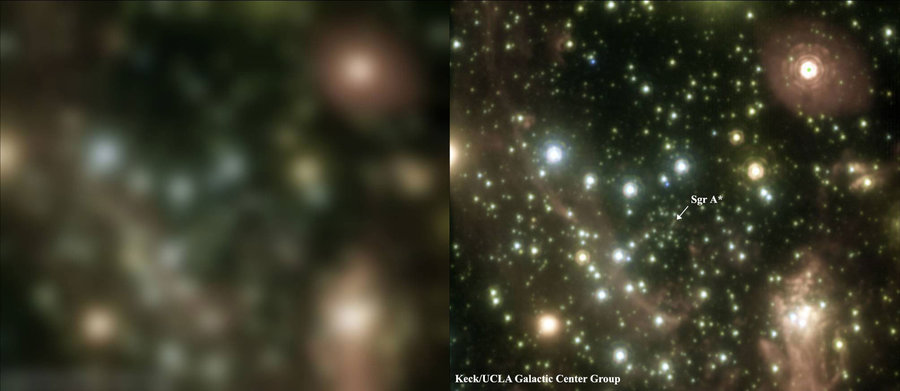
A side-by-side comparison of an image taken without and with deformable mirror systems. Courtesy of NPR.
Researchers first use a very bright reference star near the object being studied to measure blurring caused by the atmosphere. There is not always a bright enough star within reasonable distance, so astronomers often simulate the same effect by projecting a laser beam up into the atmosphere. We refer to these lasers as “laser guide stars” because they essentially act as an artificial star. Deformable mirrors are controlled in real-time to correct distortion caused by the atmosphere’s effect on wavefront. Today, this technology is used in the world’s most advanced telescopes such as the VLT.
To learn about deformable mirror products from industry leaders, visit FindLight.
[…] ← Previous […]