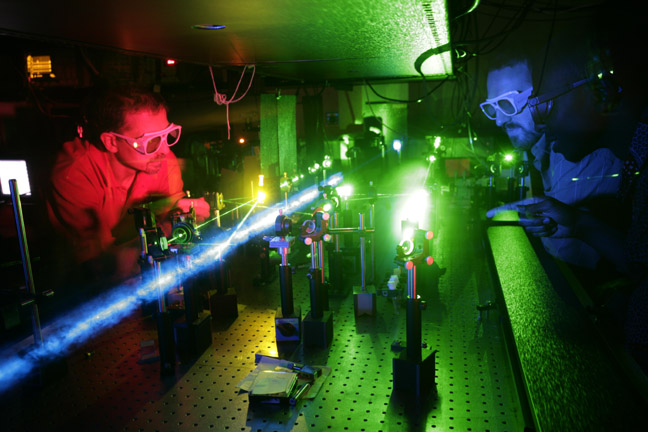
Optical parametric oscillators (OPOs) have greatly advanced the field of nonlinear optics. These devices can provide a coherent light in ranges unattainable by a standard laser. Courtesy of Lehigh University.
Introduction to Optical Parametric Oscillators
In 1961, Peter Franken and his group at the University of Michigan were the first to use nonlinear materials to produce optical harmonics. This method provided a powerful technique in generating tunable, coherent light and propelled the field of nonlinear optics. Optical parametric oscillators are light sources based on optical gain through a nonlinear medium. Whereas classic lasers rely on stimulated emission from a gain medium, OPOs use a nonlinear crystal to convert a pump beam into both a signal beam and an idler beam. The output light from an OPO system is especially useful in applications of spectroscopy.
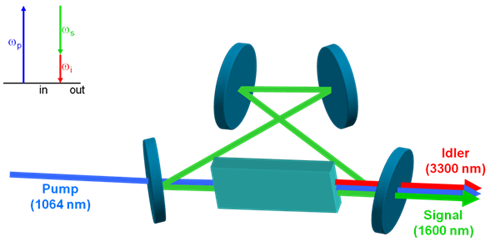
The above provides a simple schematics of a singly resonant OPO. Notice that the frequency of the pump is equal to that of the signal frequency plus idler frequency. Courtesy of Radboud University.
How do they work?
Optical parametric oscillators generate a second-order nonlinear process that involves three optical frequencies: the pump, signal, and idler frequencies. An incident laser pump beam is first focused into a nonlinear crystal. As discussed in a previous article, the light field of the pump beam will generate multiple fields due to variances in the crystal’s refractive index. The generated field with higher frequency is referred to as the signal while the lower frequency field is called the idler. The pump, signal, and idler waves interact such that the signal and idler waves amplify while the pump wave weakens. The created gain allows the resonant waves (either the signal, idler, or both) to oscillate within the resonator. The OPO is described as singly resonant when the cavity is matched to either the pump, signal or idler frequency. The system is doubly resonant when two are set to resonate together.
Types of Optical Parametric Oscillators
Q-switched Pump: The majority of OPOs are pumped with a Q-switched laser. The pulses from the laser emit on the order of nanoseconds, allowing the system to easily overcome the threshold for oscillation. This threshold is the excitation level where the laser’s output is driven by stimulated emission; at this point the system is “lasing.” Oscillators with Q-switched pumps typically produce a shorter output pulse with a larger linewidth. These systems are typically singly-resonant and pumped with an actively Q-switched Nd:YAG laser. The short produced pulses have energies in the microjoule to millijoule range and frequencies in near- to mid-infrared region.
Continuous Wave Pump: Continuous wave lasers can also pump OPOs. This pump works best with a highly nonlinear crystal gain medium such as the LiNbO3. Continuous wave pumps are best for single-frequency applications.
Mode-Locked Pump: Researchers can use mode-locked lasers to pump OPOs and produce ultrashort pulses of light. The frequency within the resonator of these oscillators matches the pulse repetition rate of the pump. This provides output pulses with high energy while requiring significantly less than 1 Watt of power for the pump.
Applications of Optical Parametric Oscillators
Spectroscopy
Optical parametric oscillators are extremely useful because they can emit light in the mid-infrared or far-infrared range. This makes them especially valuable for spectroscopy where lasers fall short. Molecules attain a characteristic vibrational spectrum. This property is especially prevalent within the mid-infrared region where a large number of molecules undergo strong vibrational transitions. Mid-IR spectroscopy, therefore, provides an important method to study molecular structure and properties. OPOs can be used as a tunable coherent light source to detect and monitor gases by measuring its absorption spectrum in the infrared.
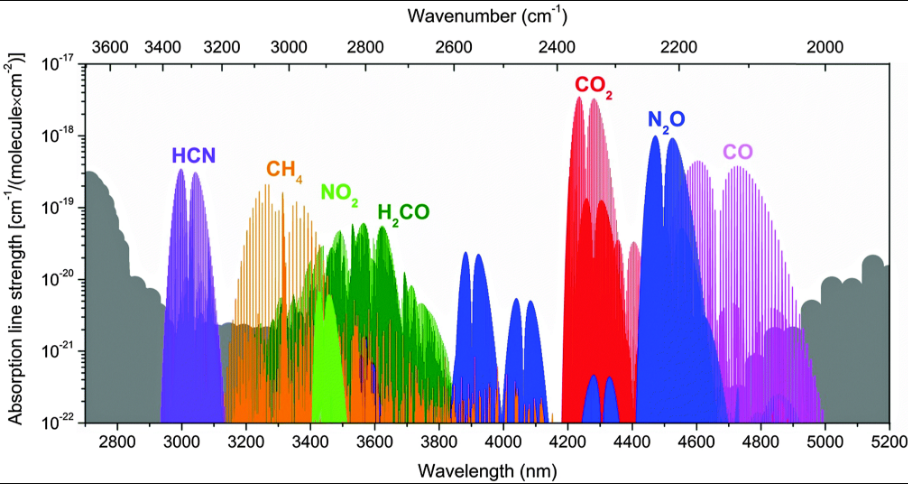
Absorption rates of various gases in the mid-infrared measured using OPOs.
Courtesy of Physical Chemistry Chemical Physics.
Trace Gas Detection
A trace gas is a gas that exists in small concentrations. Though they make up less than 1% of the atmosphere, they can be especially damaging to the environment. These trace gasses include “greenhouse gases.” Laser spectroscopy instruments are limited to monitor only a single type of gas due to their limited scanning range. OPOs in combination with spectroscopy techniques, however, prove to be much more versatile in trace gas detection. Researchers tune either a Q-switched or continuous wave optical parametric oscillators through a broad range of the mid-infrared to detect trace gases.
Biomedical Imaging
Optical microscopy is an imaging method able to examine living tissue at a resolution superior to ultrasound or MRI. On its own, however, this microscopy technique is limited in its ability to detect chemical composition of tissue structures. Researchers are now studying a nonlinear Raman scattering technique which employs an optical parametric oscillator to best identify the biochemistry of tissues. This method uses a mode-locked pump to produce strong vibrational signals for high-sensitivity imaging. To date, anti-Stokes Raman scattering microscopy has recorded images of mouse tissues with great success. The images are non-invasive, extremely high resolution, and taken in real-time. Biomedical imaging using OPOs could prove to be exceedingly useful for tissue pathology.