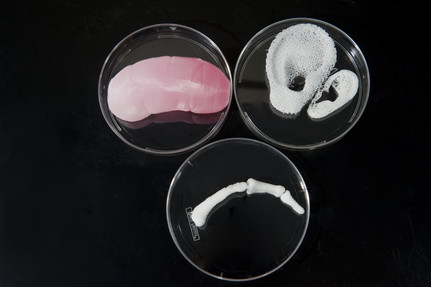
Pictured above are three real examples of 3D bioprinting results. The Wake Forest Institute for Regenerative Medicine 3D printed a human kidney, ear and finger scaffolds with a bioprinter. Photo courtesy of Joint Base Langley-Eustice
In 1984, Charles Hull placed the first building block of 3D printing technology: stereolithography. This early technique used lasers to bind polymers together in a predetermined design. Engineers used a computer to model the dimensions for each object’s design. No form of 3D printing/lithography would be possible without employing computers to specify an object’s dimensions. This first link between computer aided design and printing would pave the way for bioprinting techniques of all kinds. Early embodiment used polymer materials that quickly deteriorate, making them primarily used to design prototypes.
With the proliferation of computational power, organ printing would not be limited by design factors. The next problem that organ printing has faced is the multitude of requirements a printed organ needs to replicate its function in the body. First, a printed organ must be made from long-lasting material that doesn’t deteriorate in the body. The materials must also be able to absorb proteins, oxygen or other nutrients in the body. For these reasons, organ printing requires biological material that is compatible with the human body and won’t provoke a response from the immune system.
Stem cells or donor organ tissue are the best candidates, since they don’t trigger an immune system response. These materials can also replace traditional materials in 3D printing machines, making them good for in vivo transplants.
The Three Ways of Bioprinting
Three different forms of bioprinting exist. These include droplet based bioprinting, which is based on inkjet printing. Extrusion based bioprinting is more advanced, using a robotic system to “extrude” or force out biological material. This is most commonly used to print live cells. Laser based bioprinting is the least popular method, but it is quickly growing. It uses a pulsed laser printer to print cells with a cell-level spatial resolution. Each form has its own set of applications, advantages and disadvantages.
Droplet bioprinting is by far the cheapest, since it utilizes existing inkjet technology. Droplet printers may need modifications to house living cells and to print onto controlled platforms, but commercial printers still serve as the basis. The inkjets drop the living cells onto a stage, as if it were a piece of blank paper. As they land, the cell droplets cross-link and solidify, eventually forming a three-dimensional construct. The biggest limitation to this method is its inability to print multiple cell types. This limits droplet printing to homogeneous tissue samples, rather than forming a complete organ or cell from scratch.
Extrusion based bioprinting puts out a constant stream of filament instead of droplets. These systems are the most popular form of 3D printers. A robotic arm suspended on two axes disperses the filament. The axes enable the arm to move both up and down, as well as rotate around the object. This mechanism allows the arm to deposit filament in three dimensions. The needle that extrudes filament has a heated tip, so that the filaments are moldable and can fuse together.
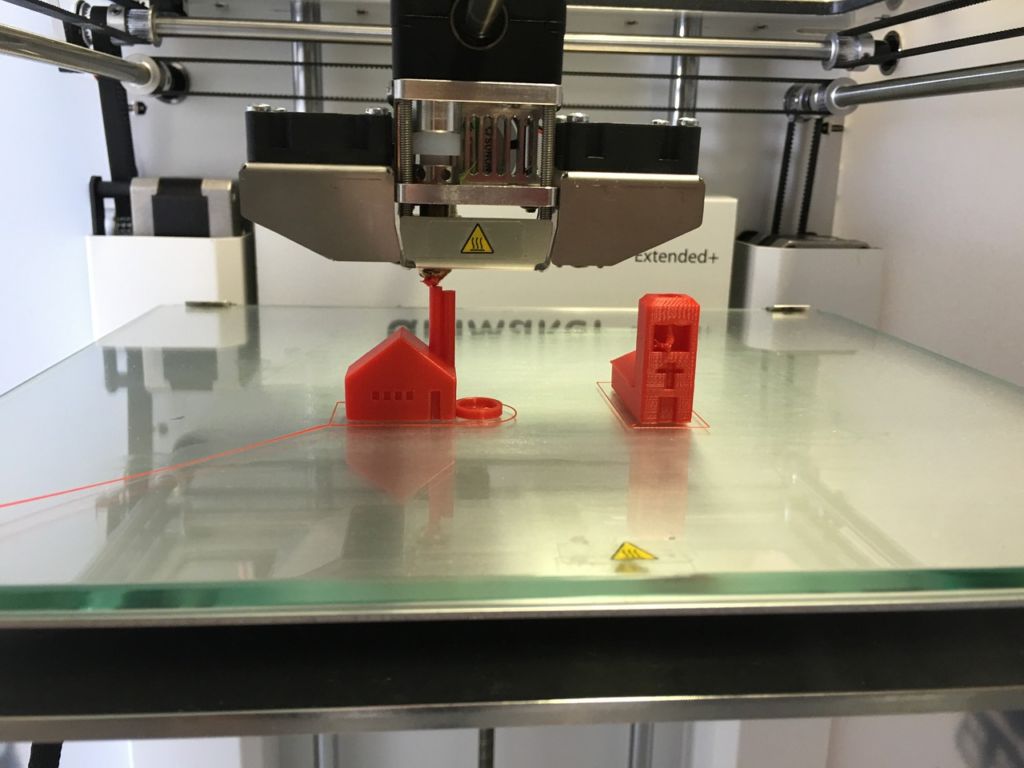
An example of an extrusion-based printer. The two needles extrude filament, while the axes on each side of the machine control the direction of the needles. As you can see, it can form a number of 3D objects on a platform using this directional control. Photo courtesy of Wikimedia Commons
Laser based bioprinting uses a printer that consists of a pulsed laser and two slides: one that can absorb energy (metallic) and one that holds the biological material. As the metallic side absorbs the laser, it forces the cells out of the biological slide. After absorbing the shock, cells shoot onto a surface where they form a 3D object in the same fashion as traditional 3D laser printing. Laser printing’s leg up on droplet and extrusion printing is its lack of a nozzle or inkjet, that is prone to clogs. However, laser printing can be costlier and has fewer established methods for bioprinting.
Are fully artificial organ transplants fact or fiction?
While it is a sensational idea, directly replacing an organ with a bioprinted copy is still far from realization. Simple organs like the bladder contain only two cell types. However, organs like the kidney, liver and heart have many different tissue types. These organs have complex filtration systems and arterial structures that must perform multiple functions. Currently, scientists employ organ printing technologies to accomplish other tasks that need replica tissue. Printing an entire organ from scratch is logistically difficult, especially when it comes to implanting. A scaffolding or frame that could provide the foundation for an organ is a more attainable goal. From there, a patient’s own organ cells can regenerate around the scaffolding, producing a nearly identical replacement.
This is commonly tested on the liver, as it is the most easily regenerateable organ. Organs like the heart, lungs and kidneys are somewhat regenerative, need stem cells in cases of severe damage. For organs with regenerative properties or that can be induced to regenerate, this offers much more promise than plugging in artificial constructions. Understanding how the body naturally replaces cells and how the effect is produced will offer an inherently biocompatible solution for organ repairs.
In cases of acute organ damage, full replacement is not always the optimal treatment. The heart is a good example of an organ that sustains heavy damage from the deterioration of one valve or artery. Myocardial tissue regeneration slows with age and in severe cases causes heart failure. Heart attacks cause massive loss of cardiac cells, making recovery even more difficult. Stem cell treatments are very effective in the heart to replace damaged cells. There are many published attempts to print scaffolds for valves and other parts of the heart. These treatments show promise in healing damage caused by heart failure, which is arguably the most widespread health problem in the world.
What other ways can bioprinting be used?
Bioprinted tissue also makes a great test subject for both drug delivery and drug testing. Typically, drug makers take this data from animals or through clinical trials. These can be cumbersome in their own right: animals require transportation and care. Clinical trials are costly and difficult to execute with regulations and safety concerns. More importantly, animal and human subjects have more variability in their responses. This gives researchers less control over the experiment as opposed to using bioprinted tissues. Bioprinted tissues construct multiple cell types, as found in complex organs. It recreates the porousness of human tissue, as well as its cell measurements.
Researchers engineer these artificial tissues to behave exactly as they would in the body, providing a better observational environment. They can study cell growth and morphology patterns in reaction to viruses or drugs. Organ scaffolds help test drug delivery methods by providing a realistic cellular response. Since the cells are from a biological sample, they have the same functions as a human organ cell. One use is the reconstruction of blood vessel lining using human cells along with a hydrogel scaffold. Scaffolding with the use of live cells is the only way to accomplish this, since blood vessels have an extraneous matrix of other arteries, capillaries and veins.
Vascular and heart diseases are key health problems in both the developed and developing worlds. This makes heart and artery scaffolding techniques a focal point for researchers. In addition, addressing extremely complex systems like blood vessels and heart valves makes a clear path for the same scaffolding techniques to be applied to simpler organs.