Fourier-transform infrared spectroscopy (or FTIR, for short) is a method of exploring the physical properties of solids, liquids, and gases. More specifically, it allows the study of the absorptive and emissive properties of materials. The choice of IR wavelengths in FTIR method is particularly useful for studying chemical bonds that connect atoms. It turns out that these bonds tend to vibrate with frequencies that correspond to infrared-wavelength light, and hence can easily be excited with such a radiation. Commonly, IR wavelength spectroscopy covers wavenumbers ranging from 200 to 4000 cm^-1
Introduction to FTIR Spectroscopy
The basic premise of any infrared or vibrational spectroscopy technique is the observation of how light is scattered or absorbed upon reaching a material. In inelastic collisions between infrared light and molecules (either at the surface of a solid material or as a gas or liquid) some collisions produce characteristic vibrations of varying modes dependent on the nature of the bond itself, but with a characteristic vibrational frequency.
While the full scope of such vibrational modes is vast, the vibrational modes can either be Raman active (i.e. light is scattered due to change in polarizability during vibration), IR active (i.e. light is absorbed due to change in dipole during vibration), or both. For this reason, Raman spectroscopy and IR spectroscopy tend to be complementary techniques, as they provide different spectra depending on the chemical bonds present in the sample. For example, ionic bonds such as C-H tend to appear as strong peaks in IR spectroscopy, and covalent bonds such as C=C tend to appear as strong peaks in Raman spectroscopy. You can read more about Raman spectroscopy and microscopy in one of our prior blog posts here.
How does FTIR Spectroscopy Work?
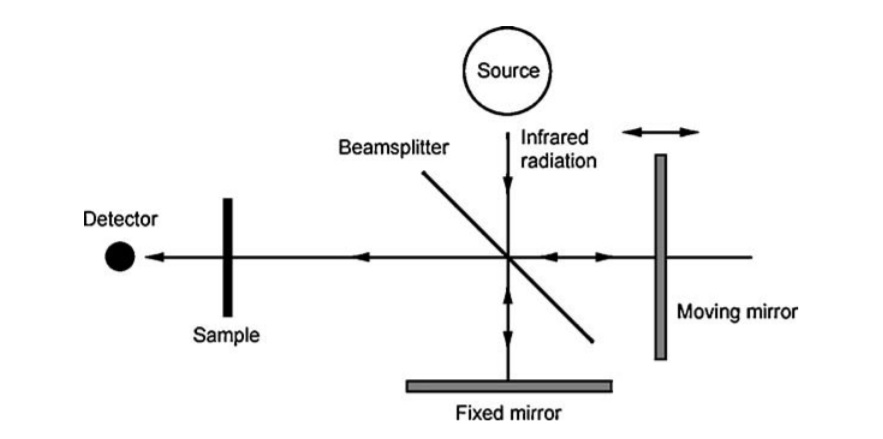
Basic schematic of Michelson interferometer, courtesy of Leng, “Materials Characterization”
The most important component of FTIR spectroscopy, and where it derives its distinction from typical infrared spectroscopy, is the Michelson interferometer. Whereas in dispersive IR spectroscopy monochromatic light sequentially irradiates a sample spanning an infrared wavelength range, FTIR spectroscopy irradiates a sample with many frequencies of IR light at once, utilizing an interferometer and post-processing of the transmitted light.
Without going too much into details, a beam source of various IR wavelength light is sent through a beam splitter, where half reaches a fixed mirror and half a mirror that moves with a constant velocity. These two split beams are then reflected and recombined (now with a path difference between the beams) to construct an interference pattern reflecting the constructive and destructive interference of the recombination. After, this interference pattern (or interferogram) is sent to the sample, and the transmitted portion of the interferogram is sent to a detector. After comparison with a reference sample beam spectrum in the detector, a Fourier transform is performed to obtain the full spectrum as a function of wavenumber.
While all of this might seem quite arcane when compared to the seemingly simple technique of sequential monochromatic irradiation, Fourier-transform spectroscopy offers a number of key benefits that have made it the dominant method of IR spectroscopy. When information from all wavelengths is collected at once, there tends to be signal-to-noise ratio reduction in the output spectra. Furthermore, the more obvious benefit of performing all wavelength measurements at once is the speed reduction when compared to dispersive wavelength techniques. However, since FTIR has been unquestioned industry standard for many decades now, it is possible that forthcoming technique breakthroughs or advances in dispersive techniques may yet catch up.
FTIR: What is it Good For?
Applications of FTIR truly run the gamut, since just about all materials are able to be analyzed by FTIR spectroscopy to provide valuable information, but it has perhaps found its most frequent use in the analysis of carbon-containing organic compounds, for which there is a comprehensive knowledge base of characteristic vibrational frequencies. As previously mentioned, chemical bonds between atoms vibrate at characteristic frequencies which have been widely tabulated, allowing for determination of the composition of samples or presence of potential contaminants by comparing the peaks of an IR spectra with tabulated characteristic frequencies.
Furthermore, quality analysis of various samples can also be determined using FTIR. For example, deviations in the characteristic spectra from a material can be indicators of oxidation or other contamination. In polymers, deviations from an expected IR spectrum can indicate a different degree of polymerization (curing) or decomposition from a reference sample. For these reasons, FTIR is widely used in such disparate applications as polymer characterization, forensic analysis, pharmaceutical analysis, and food testing.
State-of-the-Art and Future Developments
Because infrared spectroscopy is naturally diffraction-limited in the information it can obtain from any given sample, new methods have been devised to perform IR probing on the nanoscale. Nano-FTIR is a technique utilizing scanning probe microscopy and FTIR in a complementary fashion to provide infrared spectrographic information with a special resolution on the order of 10-20nm.
In Nano-FTIR, the whole FTIR setup as previously described illuminates the atomic force tip of an atomic force microscope probe with broadband infrared light, which illuminates the incoming light, creating a strong near IR field on the surface of the sample. The infrared light back-scattered from the tip of the atomic probe is then collected and an IR spectrum can then be constructed from an area on the order of tens of nanometers. A recent invention first patented in 2005, nano-FTIR has seen widespread use in probing the secondary structure of proteins, nanocomposites, and organic thin-film materials.
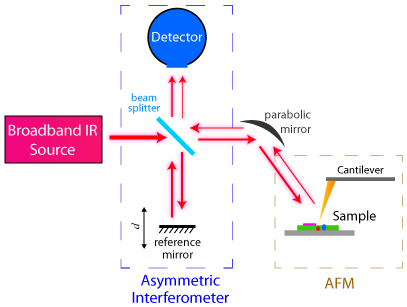
Basic diagram of Nano-FTIR setup combining atomic force microscopy and FTIR characterization techniques. Courtesy of Wikimedia commons..
While revolutionary changes in how FTIR is fundamentally operated are likely not in the cards, there are numerous domains in which FTIR technology continues to improve. The continuing development of deep learning techniques may decrease manual lookup of tabulated characteristic vibrational frequency values, while developments in IR source devices and detector devices may continue to increase efficiency. As we continue pushing the lower limits of spatial resolution in material characterization demands, it is likely that more techniques to bring IR spectroscopy principles to the nanoscale will be seen.
Comparative Analysis of FTIR with Other Spectroscopic Techniques
The landscape of spectroscopic techniques for material analysis is rich and varied, with each method offering unique insights based on its interaction with matter. While Fourier-transform infrared spectroscopy (FTIR) excels in identifying molecular vibrations and functional groups, other techniques like UV-Visible (UV-Vis) spectroscopy, X-ray diffraction (XRD), and nuclear magnetic resonance (NMR) spectroscopy provide complementary data, enabling a more comprehensive understanding of materials. This section delves into the comparative strengths of FTIR and its interplay with these prominent spectroscopic methods.
FTIR vs. UV-Vis Spectroscopy
Both FTIR and UV-Vis spectroscopy probe different aspects of molecular behavior. FTIR focuses on vibrational transitions within molecules, making it highly effective for studying molecular structures and functional groups. This specificity is particularly valuable for identifying organic compounds and assessing their chemical makeup. In contrast, UV-Vis spectroscopy examines electronic transitions, offering excellent utility in analyzing conjugated systems and determining the concentration of substances. While UV-Vis is adept at studying compounds that absorb visible and ultraviolet light, FTIR provides a more nuanced picture of molecular architecture through its sensitivity to vibrational modes.
FTIR vs. X-ray Diffraction (XRD)
XRD stands apart in its ability to elucidate crystalline structures, offering critical insights into the arrangement of atoms within a solid and identifying different phases within materials. This makes XRD indispensable in materials science and mineralogy for characterizing crystalline compounds. Conversely, FTIR’s forte lies in its broad applicability to both organic and inorganic compounds, capturing the nuances of chemical bonding and functional groups across a diverse array of materials, including those that are amorphous. This versatility enables FTIR to serve a wide range of applications, from pharmaceuticals to polymers, complementing XRD’s structural analysis.
FTIR vs. Nuclear Magnetic Resonance (NMR) Spectroscopy
NMR spectroscopy offers a profound level of structural detail, especially concerning the hydrogen and carbon framework of organic molecules. This detail aids in the precise elucidation of molecular structures, including the spatial arrangement of atoms. FTIR, while not providing the same depth of structural information as NMR, complements it by quickly and cost-effectively identifying functional groups and assessing material composition. The combination of FTIR and NMR can be particularly powerful, with FTIR screening samples for characteristic functional groups and NMR providing in-depth molecular structure analysis.
Integration and Complementary Use
The true strength of FTIR emerges when used in conjunction with other spectroscopic techniques. In multidisciplinary research and complex industrial applications, integrating FTIR with methods like UV-Vis, XRD, and NMR can furnish a holistic view of a material’s properties. For instance, in pharmaceuticals, FTIR can identify and quantify active ingredients, while NMR and XRD can provide detailed structural confirmation. Similarly, in materials science, FTIR’s analysis of organic components in composites can be complemented by XRD’s insight into crystalline matrices.
Combining these techniques allows researchers and professionals to cross-validate findings and gain a multi-dimensional understanding of materials, leading to more accurate and comprehensive analyses. This integrative approach underscores the importance of selecting the right spectroscopic tools to address specific questions, leveraging the unique strengths of each method to advance knowledge and innovation in material science.
Conclusion
The exploration of materials through spectroscopic techniques represents a cornerstone of scientific inquiry, offering a window into the molecular and structural intricacies that define their properties. Fourier-transform infrared spectroscopy (FTIR) stands as a pivotal tool in this quest, distinguished by its ability to elucidate the vibrational characteristics of molecules, thereby revealing the functional groups and bonding patterns that underpin material behavior.
The comparative analysis with other spectroscopic methods such as UV-Vis spectroscopy, X-ray diffraction (XRD), and nuclear magnetic resonance (NMR) spectroscopy has underscored the complementary nature of these techniques. Each method brings to light different facets of material composition and structure, with FTIR providing rapid, detailed insights into molecular vibrations and functional groups. The integration of FTIR with other spectroscopic approaches enhances the breadth and depth of material analysis, enabling a more comprehensive understanding that is greater than the sum of its parts.
In the dynamic landscape of material science and analytical chemistry, the continued evolution of FTIR and its integration with other techniques will undoubtedly play a critical role in advancing our understanding of materials. From the development of new materials with tailored properties to the elucidation of complex biological systems, the strategic application of FTIR and complementary spectroscopic methods will remain indispensable.
As we look to the future, the ongoing refinement of FTIR technology, coupled with advancements in complementary spectroscopic techniques, promises to expand the horizons of material characterization. The synergy among these methods will not only enhance our capability to analyze and understand materials but will also drive innovation across diverse scientific and industrial domains. In this ever-evolving field, the pursuit of knowledge continues, with FTIR and its counterparts leading the way in uncovering the molecular secrets that lie at the heart of materials.
On FindLight's photonics marketplace you can find a variety of FTIR devices and accessories as well as a series of Raman Spectrometers.