Fluorescence Microscopy is a Light Microscopy technique that utilizes fluorescence and phosphorescence to capture images of organic or inorganic structures. It is one of the most common instruments in modern day biology labs, and has come a long way since its first stages. The phenomenon known as fluorescence was first discovered in 1845 by Chemist, Sir John Herschel. He found that by shining ultraviolet (UV) light on a clear quinine solution would cause it to glow a celestial blue color. However, he called it “epipolic dispersion.”
History of Fluorescence
In 1852, a British mathematician at Cambridge University named Sir George Stokes studied fresh red algae and found a similar phenomenon. He found that the light emitted from the algae contained longer wavelengths than the transmitted UV light. He coined this effect “fluorescence” from the Latin roots fluor-spar, which mean “to flow”and “a rock.” The next big step in fluorescence history is in the early 1900’s, where fluorophores were used to stain bacteria and tissues in biology labs. This allowed biologists to research and even diagnose patients more easily, knowing which pathogens were acting in certain parts of the body. Further, in 1904 and 1911 Carl Zeiss and Reichert created the first fluorescence microscopes.
Full on Fluorescence labeling became active in the 1940’s. It is the process of binding fluorescent dyes covalently to nucleic acids and proteins for imaging purposes. In more recent times, Douglas Prasher sparked the green fluorescent protein (GFP) revolution in 1987. GFP was a new tracer molecule for proteins that did not require any additive molecules like its contemporary counterparts. GFP is utilized widely in modern day fluorescence microscopy, along with many other techniques. In this article, we will explore the multiphoton technique and wide-field technique of modern day fluorescence microscopy.
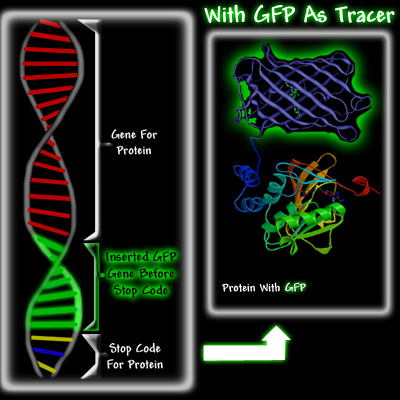
Left diagram showing normal DNA strand with GFP attached right before stop codon as protein indicator. Right: actual protein with green fluorescence. Courtesy of Conncoll.
Multiphoton Fluorescence Microscopy
Multiphoton Fluorescence Microscopy (a.k.a. two-photon excitation microscopy) is a technique that is particularly advantageous for thick specimens and 3-D imaging of living cells. For example, this technique allows embryos and even whole animals to be easily imaged. The main principle of how two-photon microscopy works is by the simultaneous (within 10 × E(-18) seconds) absorption of two photons by the fluorescent subject matter. Additionally, an important detail is that the two photons must have a wavelength that is twice as long as the wavelength for single photon absorption in the material.
For instance, fluorophores require a single photon wavelength of 350 nm to instigate fluorescence. Thus, for double photon absorption, each photon’s wavelength must be 700 nm. Another important principle behind double-photon microscopy is the quadratic relationship between the transmitted and emitted fluorescent light. In other words, the wavelength of the emitted fluorescent light is the square of the absorbed transmitted light.
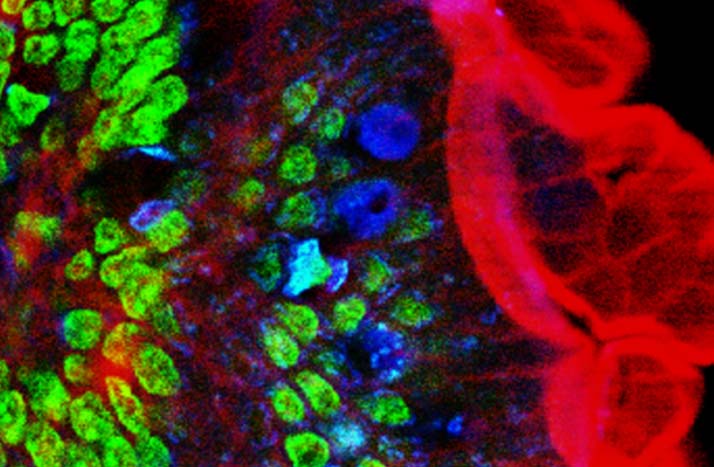
Image of mouse intestine cells produced utilizing two-photon fluorescence microscopy techniques.
Courtesy of Wikipedia.
Possible Drawbacks of Two-Photon Microscopy
A possible drawback to consider is that the setup for two-photon microscopy requires a lot of resources compared to single-photon microscopy (SPM). This is because compared to SPM, TPM requires one million times the number of photons. In other words, the two-photon absorption events do not happen as often, and an extremely high power laser must be utilized to make TPM happen. For example, among the commonly used lasers, focused mode-locked and pulsed lasers are types that can be utilized for TPM purposes.
However, only the peak of the beam pulses is powerful enough for two-photon absorption events. Thus after utilizing this kind of laser, the material ends up being in the same excited state as if a conventional (for fluorescence microscopy) powered laser was used. In addition, the material’s state would be similar to that in a single-photon experiment. Overall, the drawbacks include a great amount of power loss, but the depth of two-photon microscopy is unmatched by similar methods. Additionally, TPM is very gentle to fluorescent living cells that are sensitive to phototoxic effects of UV light.
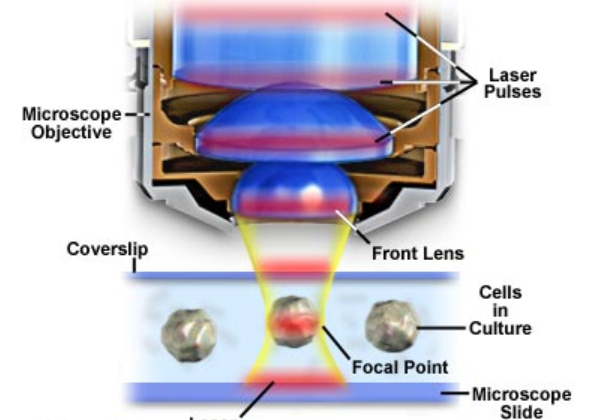
Diagram of Multiphoton Microscope setup with laser pulse shown in red.
Courtesy of Olympus.
Three-Photon Microscopy
Similar to two-photon microscopy (TPM), three-photon microscopy is another technique in the multiphoton realm. Unlike the jump from SPM to TPM, three-photon microscopy only requires about 10 times the amount of photons as TPM. Furthermore, two-photon and three-photon absorption events can occur while utilizing one laser beam. This is also advantageous because more than one type of light-absorbing fluorophore can be tested in the same experiment. One example would be with an infrared laser (1050 nm beam). Two-photon excitation can occur with green absorbing fluorophores, while three-photon absorption can occur with ultraviolet absorbing fluorophores. The particular advantage of this technique is that it works well in the deep ultraviolet spectrum. Typical microscopes run into many quality issues with wavelengths under 300 nanometers.
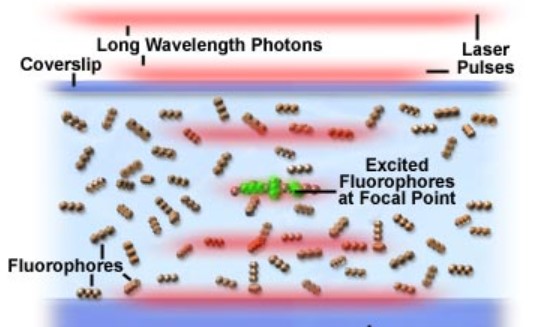
Fluorophore excitation in Multiphoton Microscopy experiment on a glass slide.
Courtesy of Olympus.
Wide-Field Fluorescence Microscopy
The second technique we will discuss here is Wide-Field Microscopy (WFM). Unlike Multiphoton Microscopy that utilizes point focused lasers, in WFM the whole sample is illuminated with light. WFM is advantageous for fast, real-time feedback and monitoring of 2-D images. Thus, WFM is perfect for imaging things like neuro-signals in living cells. It is also great for whole animals, like multiphoton microscopy, with the exception of depth limitation. Additionally, WFM can be adjusted to magnify specific cells and smaller molecules with fast temporal resolution.
The equipment setup for WFM involves a light source (commonly a mercury lamp) that produces pure white light. Next are the optical filters that filter only the wavelengths of choice from the lamp through to the sample. The filter process also involves a dichroic mirror, which reflects certain wavelengths of light and lets others pass through it. This mirror is setup to direct the right wavelengths from the lamp light source to the sample. One of the last required pieces is the microscope, which must be set to the objective that gives the wanted field of view. Finally, we have the detector or camera (typically a CCD) that picks up the fluorescent light images.
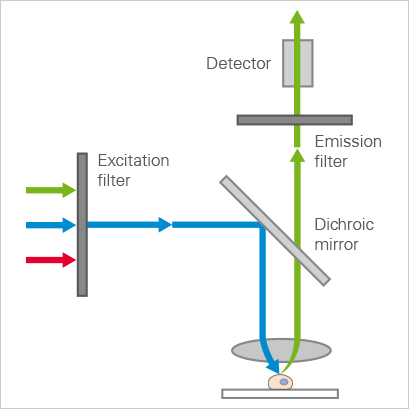
Diagram of light pathways in a widefield microscope.
Courtesy of Ibidi.
Conclusion
Fluorescence microscopy is a great tool for biologists, as it allows us to view and study living things in great detail and easily. Among the different fluorescence microscopy techniques, multiphoton microscopy and wide-field microscopy are some of the most interesting and useful. With each technique respectively, we can produce high resolution 2-D and 3-D images; from molecules and species, to whole specimens. Fluorescence microscopy continues to prove one of the top imaging techniques from its discovery to today.
Today's post was sponsored by Gentec-EO - leader in laser beam measurement solutions