We have previously discussed metamaterials in an overview regarding their uses in lens design and optical cloaking, but the metamaterial realm stretches much further than that. Today, let us dive into metamaterial usage in signals and communications–both acoustic and optical. The versatility in usage is made possible through different types and structures of metamaterials. Specific materials and structure types lend themselves well to these areas of application.
Today’s blog post is sponsored by Connet Laser– a leading manufacturer of fiber lasers and amplifiers.
Metamaterial Classification
Metamaterials are special for what they can achieve at the sub-wavelength level. Metamaterials can be classified based on their permittivity and permeability. When both permittivity and permeability are positive, the material is referred to as Double Positive. Dielectric materials fall under this category. If permittivity is negative and permeability is positive, then the medium is epsilon negative. With positive permittivity and negative permeability, the material is called µ-negative. Both negative permittivity and negative permeability materials are referred to as a double negative medium. Double negative mediums are not naturally occurring. Double negative materials exhibit physical properties that contradict the expectation. For example, compressing a double negative material will cause it to expand. A lens design that would normally be converging, if made of a double negative material, would become diverging. When permittivity and permeability are both negative, resonance can occur over a small range of frequencies.

This visually breaks down the different classifications of metamaterials. Courtesy of IntechOpen.
Metamaterial Antennas
An advantage of metamaterials is their scale. Since they work at the subwavelength/nanoscale level, devices can be miniaturized while being equally (if not more) effective. One such application is in metamaterial antenna systems. By using metamaterials, the antenna can actively bend radio waves. This yields higher efficiencies and speeds. Metamaterial substances can also increase the transmission rate, as well as direct the transmitted signals. This results in the ability to create directive, high gain antennas.
Metamaterials can enhance antenna function by using both artificial magnetic conductors (AMCs) and high-impedance surfaces (HISs). In AMCs, metamaterials are typically positioned in a way to strategically direct the radiating signals. The AMC’s purpose is to approach the performance of what a perfect magnetic conductor would be. Metamaterials can also be used in the antenna structure itself. The metamaterial structure can be designed to match the resonant frequency of the antenna. Generally, a µ-negative material would be used for this. This leads to higher gain and increased efficiency. Another option for increased gain would be to add layers of a metamaterial substrate at an optimized distance from other radiative antenna elements.
Holographic Antennas
These types of antennas function in the 500 MHz to 60 GHz range. Using holographic antennas, signals can achieve higher gain over farther distances than traditional antennas. Holographic arrays are much simpler to direct than traditional antennas. Some types of metamaterial-based antennas are completely controlled through software, switches, and/or DC biasing the array. The signal directionality can be adjusted within microseconds. This is far simpler, quicker, and condensed option for controlling antenna beams than normal. The holographic metamaterial antenna eliminates the need for additional equipment that alters the phase shift by dynamically changing the radio pattern. All of this yields a lighter, thinner, cheaper, and more efficient antenna. The operating frequency and transmission range for holographic antennas opens possibilities for wider reaching 5G networks. Holographic antennas could also potentially replace the need for fiber optic cables in some cases.
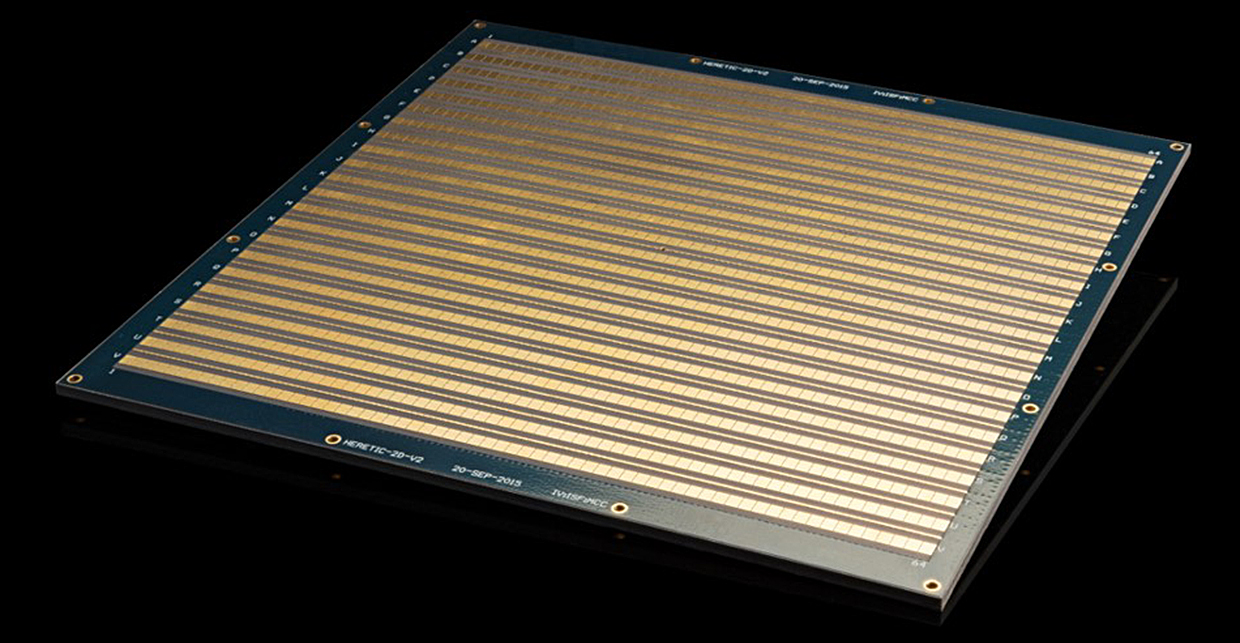
Metamaterial holographic antenna. Courtesy of Analog IC Tips.
Acoustic Metamaterials
A type of metamaterial not previously mentioned is acoustic metamaterials. The difference with acoustic metamaterials is that they are engineered to interact and manipulate the passage of phonons rather than photons. Acoustic metamaterials are made to amplify or dampen different frequencies. Acoustic metamaterials tend to be double-negative mediums. A double-negative acoustic metamaterial has both a negative mass density and negative bulk modulus. This gives the material a negative refractive index.
The potential advantages manipulating acoustic waves has not bypassed the defense industry. While cloaking was formerly mentioned for electromagnetic waves in the visible range, it was speculated there was no reason why cloaking could not be extended to acoustic waves, more specifically, to the acoustic ranges typically used for sonar.
Researchers at Duke University considered a proposal for “invisible” submarines. Instead of reflecting the signals sent out by sonar, the metamaterial shell would redirect these waves. Redirecting the signals would prevent them from being returned, thus indicating there was no obstruction (or submarine) present. For all intents and purposes, this would render the submarine undetectable. Much like the cloaks in the visible range, the acoustic cloaking devices were found to only work in two dimensions. This means that acoustic metamaterial cloaks could only obscure a submarine from another sending out sonar if—and only if—it was the same depth. Their research also assumed the surrounding medium to be different densities of water dependent on direction which poorly models the ocean. More work needs to be done until acoustic cloaking extends to three dimensions.
Another application for acoustic metamaterials is in the aerospace industry. Areas of interest include creating less noisy aircrafts. This would benefit both passengers aboard the aircraft and communities such an aircraft would fly over. The end goal would be to reduce the overall noise pollution to which aircraft contribute. The areas of highest interest to use metamaterials for noise dissipation would be areas near the engine (if not engine components) and parts of the wings. Research has indicated that creating metamaterial liners made to absorb noise from the tank of the engine from the 100-1000 Hz range would be among the most effective solutions. Suggested designs include thin films that use resonance to dampen noise while another uses a honeycomb structure, and yet another uses a metamaterial coil design to absorb sounds waves. At this juncture, designs are still in proposal and testing stage and not in practice.
What other metamaterials or applications are you familiar? Please comment below.