This white paper explores the application of ATR Probes for Harsh Environments, focusing on their critical role in optimizing process control under extreme conditions. It details the selection of materials, design considerations, and technological integrations that make these probes important tools for rigorous industrial applications.
This article is brought to you by art photonics, GmbH – offering over 20 years of experience in design, development, and manufacturing of specialty optical fibers and complex optical fiber assemblies.
Table of Contents
- Introduction
- ATR Probes for Harsh Environments: A Critical Tool for Extreme Conditions
- Unique Challenges in Harsh Environments
- Material Selection in ATR Probes for Harsh Environments
- Technological Innovations in Fiber Optics
- Interface and Integration with Process Spectrometers
- Selecting the Right ATR Probe Configuration
- Conclusion
- Recommended Literature
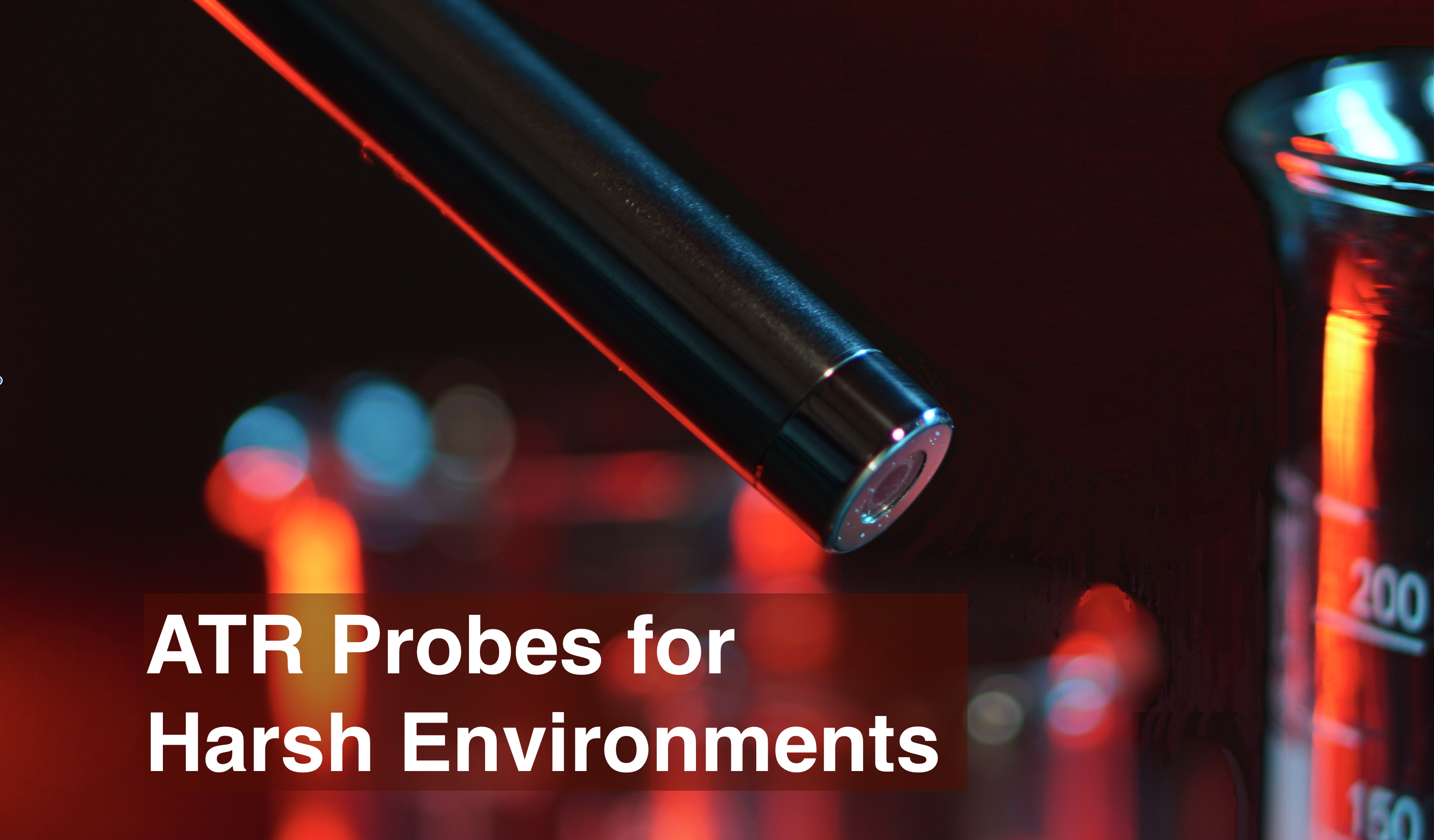
Featured: Advanced ATR Probe in Action – Engineered for Extreme Conditions. Image courtesy of art photonics GmbH.
1. Introduction
Process control in harsh environments presents unique challenges, demanding robust and reliable instrumentation capable of withstanding extreme conditions such as high temperatures, corrosive chemicals, and intense pressures. In these settings, traditional spectroscopic tools often fall short, necessitating advanced technologies tailored for durability and precision.
Attenuated Total Reflection (ATR) probes emerge as indispensable solutions in such scenarios, offering high resilience and accuracy. ATR technology utilizes a specialized optical technique where an infrared light beam is internally reflected within a crystal, interacting with a sample at the interface. This interaction provides critical data, enabling continuous monitoring and control of complex industrial processes.
Although NIR and Raman spectroscopy probes are effective for certain applications and can be more cost-effective, ATR probes excel in environments where strong vibrational spectroscopy data is needed from surfaces directly exposed to extreme conditions. ATR probes are particularly valuable in situations requiring rapid, on-the-spot chemical analysis with minimal sample preparation, thereby offering distinct advantages in terms of operational efficiency and data reliability.
The ability to operate reliably under such demanding conditions positions ATR probes as crucial tools for industries ranging from pharmaceuticals to petrochemicals, where process optimization can hinge on the accurate and immediate analysis of diverse substances.
2. ATR Probes for Harsh Environments: A Critical Tool for Extreme Conditions
ATR (Attenuated Total Reflection) probes operate on the principle of evanescent wave spectroscopy. The core component, typically made from robust materials like diamond or zirconium oxide (ZrO2), serves as the ATR crystal. This crystal is in direct contact with the sample, allowing the infrared light to reflect internally and interact with the sample at the interface. The depth of penetration of the evanescent wave into the sample is minimal, often only a few microns, which is crucial for obtaining surface-specific information without damaging the sample.
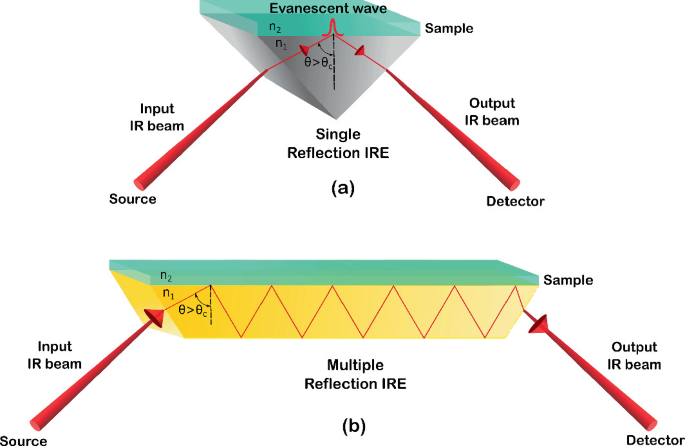
Schematic of the attenuated total reflection (ATR) crystal for ATRIR measurements. (a) The closeup of the input IR beam and the generated evanescent wave. (b) Geometry of multiple internal reflection within the ATR crystal adjacent to the sample. Image courtesy of Harsharan Kaur, Bhawna Rana, Deepak Tomar, Sarabjeet Kaur & Kailash C. Jena from their article ‘Fundamentals of ATR-FTIR Spectroscopy and Its Role for Probing In-Situ Molecular-Level Interactions,’ published in the Journal of Modern Techniques of Spectroscopy on 02 April, 2021.
The utilization of ATR probes designed for harsh environments provides several advantages. Firstly, their robust construction allows them to withstand conditions that would degrade conventional spectroscopic tools. This includes resistance to extreme temperatures, which can reach up to 300°C, and pressures up to 300 bar, as well as exposure to aggressive chemicals and corrosive environments. Secondly, ATR probes offer rapid and direct measurements without the need for extensive sample preparation, facilitating real-time process monitoring and control. This immediacy and accuracy in data collection are vital for maintaining the integrity and efficiency of industrial processes under extreme conditions. Additionally, some ATR probes, such as those supplied by art photonics, are designed to be compatible with a variety of spectrometric systems, including FTIR and NLIR mid-infrared spectrometers as well as Quantum Cascade Laser (QCL) systems, enhancing their applicability across diverse technological platforms.
3. Unique Challenges in Harsh Environments
Harsh industrial environments often subject spectroscopic equipment to extreme conditions that can rapidly degrade their performance and structural integrity. Among these challenging conditions are:
- Extreme pH Levels: Equipment used in environments with very high or very low pH values faces significant chemical aggression. Specifically, a very high pH indicates a strong base (alkaline solution), while a very low pH signifies a strong acid. Both conditions can corrode standard materials used in probe construction due to their caustic nature.
- High Temperatures: Temperatures exceeding 200°C are common in many industrial processes, such as petrochemical refining and energy production. These conditions demand materials that maintain their mechanical and optical properties at elevated temperatures.
- High Pressures: In applications like deep-sea exploration and hydraulic fracturing, pressures can exceed hundreds of bars, necessitating probes that can withstand such forces without compromising the integrity of the optical path.
- Electromagnetic Interference: Exposure to microwave electromagnetic fields and gamma radiation can alter the physical properties of the probe materials and interfere with the accuracy of spectral data.
- Chemical Aggression: Beyond pH-related issues, harsh chemicals, including organic solvents, oxidizers, and other reactive substances, can also interact with the materials used in probes. This includes reactions that are not necessarily related to the acid-base spectrum, such as solvent effects, oxidation, and reduction processes. Such chemical interactions can lead to deterioration or failure of the equipment, a concern that is particularly critical in industries like chemical manufacturing and pharmaceuticals.
The selection of materials for ATR probes in such environments is thus crucial. Not only must these materials resist physical and chemical degradation, but they also need to be compatible with the substances they analyze, avoiding any reaction that could taint the sample or the probe. Ensuring material compatibility extends the lifespan of the probes and ensures consistent, reliable measurements essential for process optimization.
4. Material Selection in ATR Probes for Harsh Environments
Choosing the right materials for ATR probes is crucial to ensure their performance and longevity under extreme conditions. The selection covers crystals, shaft materials, and sealing components, each chosen for their unique properties:
Crystals:
- Diamond: Known for its exceptional hardness and chemical inertness, diamond is the premium choice for the harshest conditions, resisting scratches and chemical attacks, while offering superior optical clarity.
- Zirconium Oxide (ZrO2): Excellent for high temperature and caustic environments due to its thermal stability and resistance to acid and alkali corrosion.
- Germanium (Ge): Preferred in applications involving infrared light due to its high refractive index, although less durable under extreme chemical conditions.
- Silicon (Si): Offers good infrared transmission but is limited by its lower hardness and susceptibility to harsh chemicals.
- Sapphire (Al2O3): Highly valued for its excellent optical properties and substantial chemical resistance, sapphire is particularly suitable for environments where optical clarity and resistance to scratching are paramount. Its robustness against a wide range of chemicals and good thermal stability make it an ideal choice for many demanding applications.
Shaft Materials:
- PEEK (Polyether Ether Ketone): Offers excellent chemical resistance and mechanical properties at high temperatures, suitable for biomedical and pharmaceutical applications.
- Stainless Steels (SS316, SS316L, SS304, SS304L): Provide good corrosion resistance; SS316L and SS316 are particularly valued in marine applications for their greater resistance to chloride exposure.
- Hastelloy C22 and C276: These nickel alloys are renowned for their outstanding resistance to severe corrosive environments, including oxidizing and reducing media.
Sealing Materials:
- PEEK: Again, its robustness in high-temperature and corrosive environments makes it a top choice for sealing applications.
- Teflon: Known for its inertness, Teflon seals provide excellent resistance to a wide range of chemicals, making it ideal for use in chemical processing industries.
- Gold: While not commonly used for full seals, gold is often used in applications requiring extremely high corrosion resistance and excellent thermal conductivity.
The integration of these materials into ATR probes ensures that the instruments can withstand the physical and chemical stresses of harsh environments, maintaining accuracy and reliability in their measurements.
5. Technological Innovations in Fiber Optics
Advancements in fiber optic technology have significantly enhanced the durability and performance of spectroscopic instruments, particularly in challenging environmental conditions. These innovations include the development of specialized fibers designed to withstand harsh conditions and improve spectroscopic accuracy.
Unique Fiber Solutions:
- Chalcogenide Fibers: Made from materials with excellent mid-infrared transmission properties, chalcogenide fibers enable highly sensitive detection of chemical compounds even under extreme conditions such as high temperatures or in reactive chemical atmospheres.
- Hollow Waveguide Fibers: These fibers feature a hollow core with a reflective inner surface, minimizing light-material interaction. This design is advantageous for transmitting high-energy light or protecting the fiber from corrosive gases, which is crucial in high-power density applications.
- Polycrystalline Infrared (PIR) Fibers: PIR fibers stand out for their ability to transmit light up to 16 micrometers. This feature makes them particularly suitable for applications requiring high infrared light transmission where traditional glass fibers might fail.
- Fluoride Fibers: Excellent for transmitting light in the mid-infrared spectrum, fluoride fibers offer high transmission rates and are resistant to a wide range of chemicals, making them suitable for harsh environmental conditions where chemical stability and optical clarity are required.
Benefits of Specific Fibers:
- Chalcogenide Fibers: Chalcogenide fibers are essential for transmitting infrared light in mid-infrared spectrometers that operate in harsh environments. Although the accuracy of chemical analysis largely depends on the spectrometer itself, these fibers are pivotal in ensuring consistent transmission of infrared light where traditional glass fibers would fail. This capability preserves the integrity of the spectroscopic data, which is crucial for diligent process control.
- Hollow Waveguide Fibers: Unlike solid-core fibers, hollow waveguide fibers feature a hollow core with a reflective inner surface, which reduces interaction between the light and the fiber material. This design minimizes degradation from high-energy or corrosive light interactions, thus reducing maintenance needs and enhancing the longevity of the instrument. While these fibers are generally used within the probe, they are integral in ensuring that the probe’s performance does not diminish due to internal fiber damage.
- Polycrystalline Infrared (PIR) Fibers: Polycrystalline Infrared (PIR) fibers offer a significant advantage in transmitting light deep into the infrared spectrum (16 um), reaching wavelengths that fluoride and chalcogenide glasses cannot. This makes them particularly valuable for applications requiring deep infrared transmission. Compared to Hollow Waveguides (HWG), which are also used for mid-IR transmission, PIR fibers exhibit greater flexibility, providing a versatile solution for various demanding optical applications.
- Fluoride Fibers excel at transmitting light in the mid-infrared spectrum, crucial for detailed chemical analysis. They offer high transmission rates and are resistant to a wide range of chemicals, making them suitable for harsh environmental conditions where chemical stability and optical clarity are essential. Their resistance ensures that spectroscopic measurements remain precise and reliable even under adverse conditions.
Use Cases:
- Petrochemical Industry: In a petrochemical plant, the use of chalcogenide fibers for remote sensing can help monitor hydrocarbon levels continuously at multiple stages of the process, improving operational safety and efficiency. These fibers, ideal for their high sensitivity and resistance to harsh conditions, are typically employed in configurations where the proximity of the sensing equipment to the monitored processes allows for effective data transmission without the need for extremely long fiber runs, unlike applications that might utilize NIR probes with longer extension capabilities.
- Biomedical Applications: PIR and Hollow waveguide fibers can also be utilized in non-invasive cancer diagnostics to deliver high-intensity infrared radiation, achieving targeted treatment with minimal damage to surrounding healthy tissues.
- Pharmaceutical Industry: Chalcogenide fibers are critical in pharmaceutical manufacturing, where precise monitoring of chemical compositions and reactions is essential. Their ability to withstand harsh environments enables reliable real-time analysis, crucial for maintaining product quality and adherence to safety standards.
- Extrusion Processes: Hollow waveguide fibers are valuable in the extrusion industry, particularly in monitoring and controlling the quality and consistency of extruded materials. Their durability and resistance to high temperatures and corrosive materials make them ideal for real-time monitoring and control in these harsh production environments.
These fiber optic advancements demonstrate the use of smart designs in spectroscopic technology to meet the high standards of industries that require precision and reliability.
6. Interface and Integration with Process Spectrometers
The successful deployment of ATR probes in industrial settings heavily relies on their seamless integration with process spectrometers. This integration is facilitated through a variety of connection interfaces, each designed to ensure robust and reliable communication between the probe and the spectrometer systems.
Connection Interfaces:
- Fiber Optic Connectors: These are commonly used to link ATR probes with spectrometers, allowing light to be transmitted efficiently from the probe to the spectroscopic analysis equipment. Common types include SMA, ST, FC connectors, and, for specific models like the Bruker MATRIX-F and MATRIX-MF, BQC connectors. Each type is chosen based on their alignment accuracy and integration requirements.
- Electronic Interfaces: For probes that require power or need to transmit electronic data, interfaces such as USB, RS-232, or Ethernet may be employed. These interfaces support not only data transfer but also enable the control of probe parameters remotely.
- Thermocouple Integration: Some ATR probes are also equipped with a thermocouple to measure the temperature at or inside the probe’s tip. This feature is essential for applications where precise temperature monitoring is critical, ensuring that the probe operates within its optimal temperature range and enhances the reliability of the spectroscopic data.
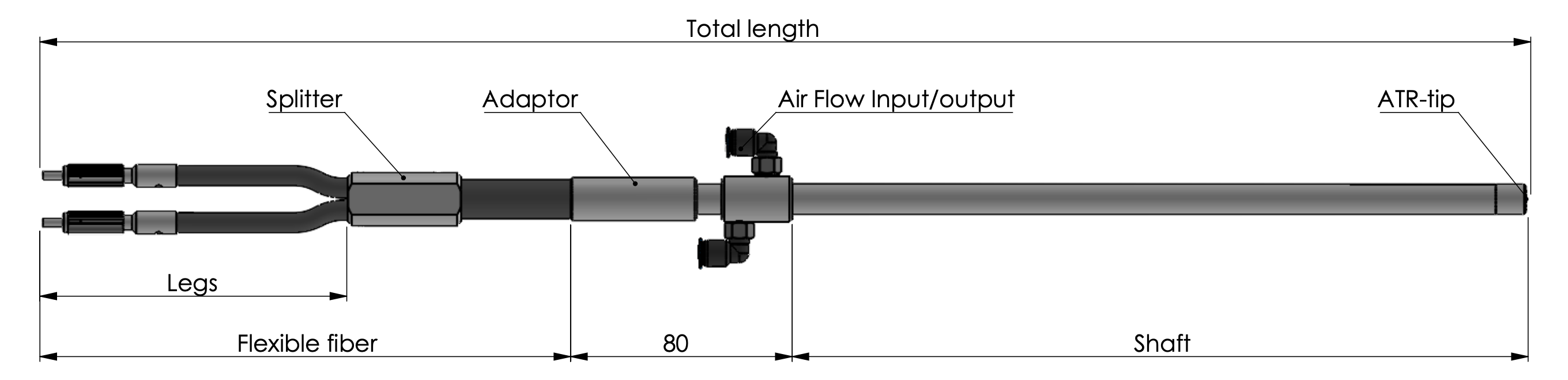
Schematics of a High Temperature ATR-Probe depicting the fiber optic legs, fiber splitter, adaptor and air flow input/output ports. Image courtesy of art photonics, GmbH.
Ease of Installation and Maintenance:
- The design of these interfaces typically emphasizes ease of installation, allowing for quick setup and integration without needing extensive technical expertise. This is critical in industrial environments where downtime for installation or maintenance must be minimized. Additionally, some of of these probes are available with specific flanges that facilitate direct connection to pipeline systems, further simplifying the installation process in complex industrial setups.
- Maintenance of these systems is also streamlined by the use of modular components. For instance, sterilizable ATR probes designed for bioreactors feature interchangeable parts that can be easily replaced or upgraded without disrupting the entire system. This modularity is particularly valuable in environments requiring stringent hygiene and maintenance protocols. However, it is important to note that not all ATR probes offer this level of interchangeability; it is generally limited to specific models tailored for particular applications.
The thoughtful design of connection interfaces and the emphasis on easy maintenance ensure that ATR probes can be quickly integrated and remain operational with minimal downtime, supporting continuous industrial processes even in demanding conditions.
7. Selecting the Right ATR Probe Configuration
Choosing the optimal ATR probe configuration is crucial for maximizing performance and ensuring reliability in specific applications. The following selection process involves understanding the unique demands of each operational environment and aligning them with the appropriate probe features.
Guidelines for Choosing the Appropriate Probe Configuration:
- Assess Environmental Conditions: Begin by identifying the operational extremes, such as maximum temperatures, pressures, and chemical exposure, which the probe must withstand. For instance, if operating temperatures reach up to 250°C, select materials like diamond, silicon or ZrO2 for the crystal. While diamond and ZrO2 are known for their exceptional resistance to high temperatures and harsh chemical environments, silicon also performs adequately up to this temperature threshold, making it a viable option depending on the specific requirements of the application.
- Evaluate Application Requirements: Consider the type of spectral analysis required. For applications requiring high signal-to-noise ratio (SNR) in specific spectral ranges, a diamond crystal might be necessary due to its broad spectral range and high refractive index. While diamond is not typically associated with high resolution for complex mixtures, its exceptional hardness and chemical inertness make it suitable for demanding environments where durability and a stable signal are crucial.
- Consider Installation Constraints: Space limitations or specific mounting requirements can dictate the design of the probe shaft and housing. Compact designs are available for constrained spaces.
Decision Matrix and Flowchart:
- Decision Factors: Include environmental resilience, spectral range, chemical compatibility, and physical constraints. Each factor is rated based on its importance to the operational context.
- Flowchart Implementation: Start with environmental conditions, followed by chemical compatibility, then move to spectral requirements, and finally address any physical installation constraints.
- Example Metric: Probes used in environments with volatile chemicals should be evaluated for their chemical and temperature resistance. These evaluations often involve standardized tests to determine a durability rating, which quantifies the probe’s ability to withstand harsh conditions. For example, a rating of 8 out of 10 might indicate high resistance to corrosive substances and stable performance at elevated temperatures, based on specific industry standards or testing protocols.
The decision matrix simplifies the selection process, offering a visual tool that aligns various probe characteristics with environmental and operational requirements. This systematic approach ensures that users can objectively compare options and make informed choices tailored to their specific needs.
Selecting Materials for ATR Probes
When choosing materials for Attenuated Total Reflection (ATR) probes, it is essential to consider the specific requirements of your application. The table below provides a comprehensive guide to various ATR probe materials, their shaft diameters, spectral ranges, fiber types, and operating conditions such as pressure and temperature. This information will help ensure you select the most suitable probe material for your spectroscopic needs, particularly in demanding environments.
Tip Material | Spectral Range (cm⁻¹ or nm) | Sealing | Fiber Type | Pressure | Temperature | pH Range |
---|---|---|---|---|---|---|
Diamond | 600 – 1900 cm⁻¹ | PEEK | PIR1 900/1000 | 200 Bar | -150 to +140 °C | 0 – 14 |
Silicon | 600 – 3100 cm⁻¹ | PEEK | PIR 900/1000 | 100 Bar | -150 to +140 °C | 1 – 10 |
Germanium | 600 – 3100 cm⁻¹ | PTFE | PIR 900/1000 | 10 Bar | -150 to +90 °C | 1 – 8 |
Cubic Zirconia | 1550 – 9000 cm⁻¹ | PEEK | CIR2 500/550 | 100 Bar | -150 to +90 °C | 1 – 14 |
Cubic Zirconia | 400 – 2200 nm | PEEK | NIR3 600/660 | 100 Bar | -150 to +200 °C | 1 – 14 |
Sapphire | 260 – 1300 nm | PEEK | UV4 600/660 | 100 Bar | -150 to +200 °C | 3 – 12 |
- PIR: Polycrystalline Infrared
- CIR: Chalcogenide Infrared
- NIR: Near-Infrared
- UV: Ultraviolet
8. Conclusion
ATR probes are indispensable for reliable process control in harsh environments using spectrometric methods. Their robust design, tailored material choices, and advanced technological integrations ensure that they meet the rigorous demands of extreme conditions. Whether facing high pressures, aggressive chemicals, or extreme temperatures, these probes provide accurate and consistent data essential for optimizing industrial processes.
The versatility of ATR probes lies in their ability to withstand and perform under extreme conditions without compromising the integrity of the measurements. By selecting appropriate materials for the probe tips and sealing mechanisms, such as diamond, silicon, germanium, and cubic zirconia, industries can ensure that their probes are suited to the specific chemical and physical environments they will encounter. Each material offers unique advantages, such as resistance to high pressures and temperatures or specific spectral ranges, making them suitable for various applications from petrochemical analysis to pharmaceutical manufacturing.
Additionally, the integration of advanced fiber optic technologies enhances the performance of ATR probes. For instance, the use of PEEK and PTFE sealing materials provides excellent chemical resistance, while different fiber types like PIR, CIR, and silica fibers for NIR, and UV enable precise spectral measurements across a wide range of wavelengths. These features are crucial for applications that require continuous monitoring and control, ensuring process stability and product quality.
In conclusion, the careful selection of ATR probe materials and designs is vital for achieving reliable and accurate spectroscopic analysis in harsh environments. By leveraging the strengths of various materials and advanced technological components, ATR probes offer a robust solution for process control, ensuring that industries can maintain efficiency, safety, and quality in their operations. As technology advances, these probes will continue to play a critical role in meeting the ever-evolving demands of industrial processes, driving innovation and improvement in a wide range of fields.
9. Recommended Literature
- Goncharov, A. F., & Crowhurst, J. C. (2005). Raman Spectroscopy under Extreme Conditions. Journal of Low Temperature Physics, 139(6), 727–737. https://doi.org/10.1007/s10909-005-6283-7.This article discusses Raman measurements of various materials under high temperature and high pressure in a diamond anvil cell. It presents Raman spectra of cubic boron nitride up to 40 GPa and 2300 K, high-pressure spectra of a new noble metal nitride, and high-temperature spectra of pure nitrogen up to 39 GPa and 2000 K. The study also examines changes in the intramolecular potential with pressure.
- Kimura, S., Nishi, T., Takahashi, T., & Hirono, T. (2003). Infrared spectroscopy under extreme conditions. Physica B: Condensed Matter, 329-333, 1625-1626. https://doi.org/10.1016/S0921-4526(02)02431-6.This article discusses the construction of a magneto-optical microspectroscopy apparatus in the infrared region using synchrotron radiation at SPring-8. The apparatus combines an infrared microscope with low temperatures and high magnetic fields to investigate the electronic structure of tiny materials under extreme conditions. The study presents the specifications of the apparatus and recent results from an organic superconductor.
- Miele, P., Snead, K., Zakhireh, N., Homa, D., Pickrell, G., & Risch, B. G. (2020). Optical Fiber Reliability in Harsh Environments. Paper presented at the 2020 International Wire and Cable Symposium. https://doi.org/10.1016/S0921-4526(02)02431-6.This conference paper discusses the mechanical reliability of optical fibers used in harsh environments, such as high pressure and temperature. The study highlights the importance of coating systems for the fibers’ longevity, presenting data on dynamic fatigue and tensile strength. Results indicate the reliability of different coatings under various conditions, essential for selecting appropriate systems for specific environmental deployments.
- Piccolo, A., Delepine-Lesoille, S., Friedrich, E., & Aziri, S. (2019). Durability and Mechanical Properties of Optical Fiber Strain Sensing Cable for Structural Health Monitoring in Harsh Environment. Paper presented at the 12th International Workshop on Structural Health Monitoring, Stanford. https://doi.org/10.12783/shm2019/32291.This conference paper discusses the durability and mechanical properties of optical fiber strain sensing cables used in harsh environments, such as those found in nuclear facilities. The study includes mechanical and thermal tests on a custom strain sensing cable before and after irradiation up to 500 kGy. The results highlight the importance of proper calibration and the moderate impact of radiation on the cable’s performance, emphasizing the suitability of optical fiber sensors for structural health monitoring in challenging conditions.