Previously, we discussed a technique for producing high resolution maps of neural activity in the brain called Optical Recording of Intrinsic Signal, or ORIS. Conventional methods for performing ORIS measurements involved mounting a CCD or CMOS camera sensor over the exposed brain of an animal or patient with the sensors measuring localized changes in reflectivity due to cerebral blood flow. Obviously this is not an ideal setup for performing continuous measurements over long periods of time. In order to produce a clinical alternative to electronic measurements of neural signal using surgically implanted electrocorticography (ECoG) arrays, researchers have been focusing on developing implantable sensors capable of performing ORIS measurement of seizure activity over the surface of the cortex.
A rudimentary sensor, which we discussed in a recent blog post, was demonstrated in 2010 and combined a discrete commercial LED and photodetector attached to a flexible circuit. Despite the simplicity of the design and fabrication, the device demonstrated high signal to noise detection of seizures in the brain by measuring large changes in reflectivity due to increased cerebral blood flow. In this article, we will discuss recent iterations on the implantable sensor design by a team of scientists at Columbia University and Weill Cornell Medical College. Their innovation further improves the prospect of a fully implantable system capable of performing sub-millimeter localized detection of seizures in the brain. The first of their inventions is a device that uses organic LEDs (OLEDs) and organic photodetectors to create an ultra-thin and ultra-flexible device. The second device uses micro LEDs with high optical performance.
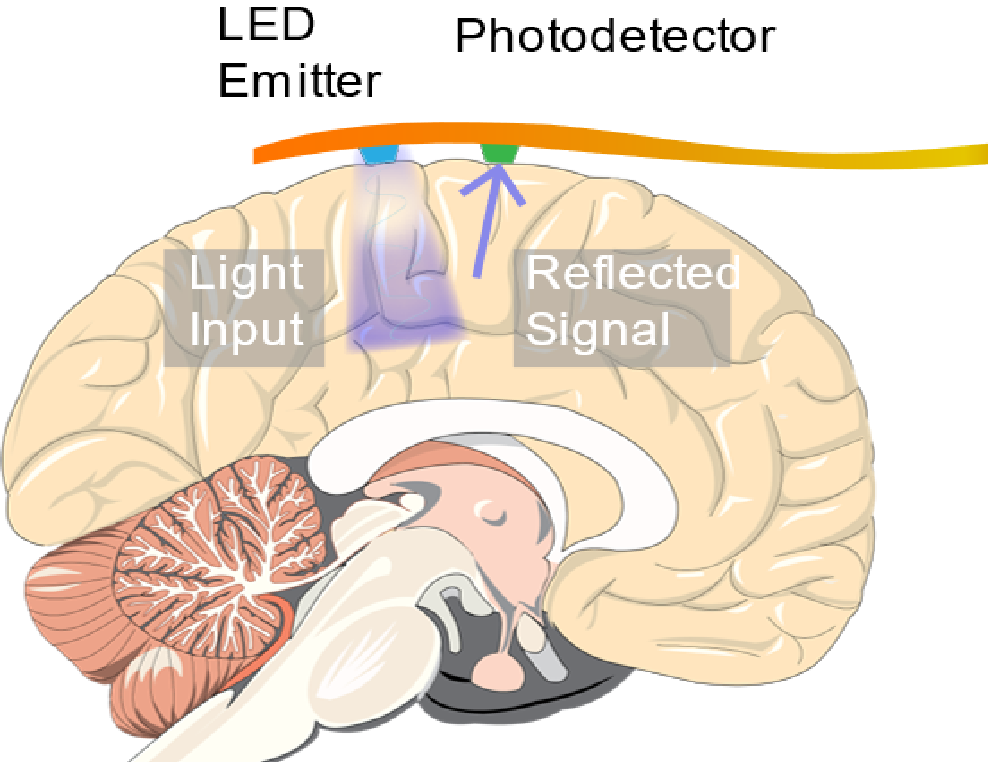
An implantable ORIS sensor consisting of an LED and photodetector mounted on a flexible circuit was used to detect seizures occurring in live mice.
What Makes a Medical Device Implantable?
According to the US Food and Drug Administration, a medical device is “…any product that does not achieve its purposes by chemical action or metabolization.” This broad definition encompasses wide range of materials and designs ranging from tongue depressors to surgical lasers. Additionally, medical devices are given classification starting from class I to Class III based on the impact and risk of treatment by the device. For instance, a neural implant device which requires invasive surgery for attachment/installation is typically considered a Class III medical device, with the strictest level of regulation. These devices face the maximum amount of scrutiny to determine whether they are safe and effective to use.
Characteristics of a high performing implantable sensor include but are not limited to: flexibility for comfortable attachment/implant, biocompatibility of materials, low harmful radiation and heat generation, safe power delivery, and practical operational lifetimes. The design of the original device that we discussed in our prior blog post featured relatively large and bulky components which limited density of sensors which could be packed onto a device. Efforts to improve this design were focused on decreasing the component size and increasing the flexibility of the device.
OLED-Based Implantable Sensor
In 2015, the research team at Columbia University and Weill Cornell Medical College demonstrated an ultra-thin and flexible device based on OLEDs and organic photodetectors at the International Electron Devices Meeting. The device was made by fabricating OLEDs and organic photodetectors directly onto a flexible substrate with in-house thermal evaporation techniques. The substrate used in this experiment was parylene-c. Parylene-C was chosen for its optical transparency at visible wavelengths, known biocompatibility, and the ability to fabricate extremely thin free standing films under 2 µm thick. Another 2 µm thick layer of parylene-c was added on top of the OLED and organic photodetector layers to provide encapsulation of the moisture and oxygen sensitive organic components against the humid environment of the brain, as well as a biocompatible interface with the brain.
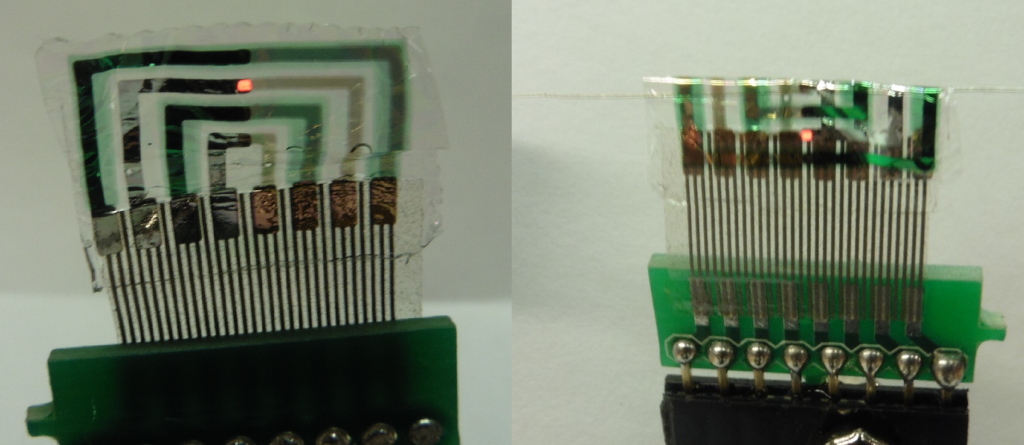
The OLED-based implantable sensor (left) is made using a parylene-c substrate. The device can be bent around a radius of 0.08 mm without damage (right).
The device, with total thickness under 5 µm, had high flexibility demonstrated by bending around curves under 0.08 mm in radius. This allowed carrying out experiments on live animals, where the device was attached to the exposed brain of a rat and successfully used to detect seizures. Green light emitting from the OLED was reflected from the surface of the brain while the photodetector was connected to a transimpedance amplifier, recording the changes in reflectivity driven by the cerebral blood flow. With a peak emission wavelength of 540 nm and an operating luminance of around 100 cd/m2, the OLED based device was able to detect the heart rate, respiration rate, and slow sinusoidal oscillations due to healthy neural activity. After epileptic like seizures were simulated through drugs, the implantable sensor was able to detect seizures occurring in real time with high accuracy and signal-to-noise.
Micro LED-Based Implantable Sensor
In a 2017 article in Advanced Materials Technologies, the researchers published developments on another type of implantable optical imaging sensor capable of performing detection of seizures using ORIS. This device used micro LEDs (surface mounted LEDs with total package sizes under 1 mm and high efficiency) instead of OLEDs and organic photodetectors to perform ORIS measurements. An array of commercial micro LEDs were mounted on a flexible polymer substrate made of polyimide. Polyimide in this case was used for its compatibility with the high temperature and chemical processing necessary to create the circuitry and bond the micro LEDs. After the micro LEDs were adhered to the substrate, a layer of parylene-c was applied for encapsulation and biocompatible interface.
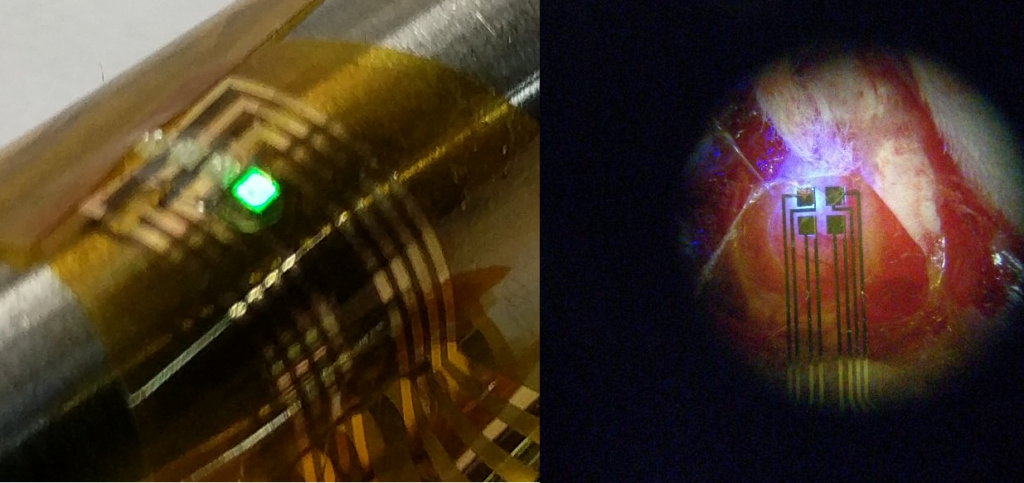
Micro LEDs were used to optically detect seizures. The flexible optical device is shown on the left with a green-emitting LED. The photo on the right shows the device performing seizure detection on a rat’s brain.
A unique aspect of the micro LED array was the use of the Mims effect (first discovered by famed citizen scientist Forrest Mims) to operate the micro LEDs as bi-directional emitter/detectors. In other words, the micro LEDs in the array could be switched between two operating modes where they either emitted light like a standard LED or sensed light like a photodetector. This configuration enables a highly versatile design with a large number of allowable geometric array configurations and a reduced number of required components.
When implanted on a live rat, seizures were detected with a high signal-to-noise ratio over 20 dB. While the published results from the team at Columbia and Cornell Weill only included devices with up to two detectors, the researchers were able to show localized effects of seizures on the cerebral blood flow at two different regions under 1.5 mm apart. A fully arrayed device of this nature could represent a hallmark in high resolution neural imaging using an implanted medical device. It is conceivable that a fully arrayed device using smaller micro LEDs might even achieve resolutions well under 1 mm x 1 mm and approaching the 100 µm x 100 µm limit of CCD/CMOS sensors.
QDs for Color Conversion
One challenge with using commercial micro LEDs is the limited selection of wavelengths, that could be instrumental in detecting oxygenation and other important signals in the blood. To address this limitation and expand the available wavelengths for performing ORIS measurements, the research team devised a method of converting blue light into longer wavelengths using quantum dots. Quantum dots (QDs), semiconducting nanocrystals, were embedded into a polymer resin made of poly (methyl methacrylate) (PMMA) and coated over individual micro LEDs using pneumatic printing. The QD-PMMA composite absorbed and down-converted the re-emitted light into a longer wavelength of choice (the re-emission wavelength is determined by the size of the QD). QDs make an attractive choice for this application for their narrow wavelength light emissions. The team demonstrated seizure detection with similar performance using micro LEDs with the QD-Polymer composite coatings.
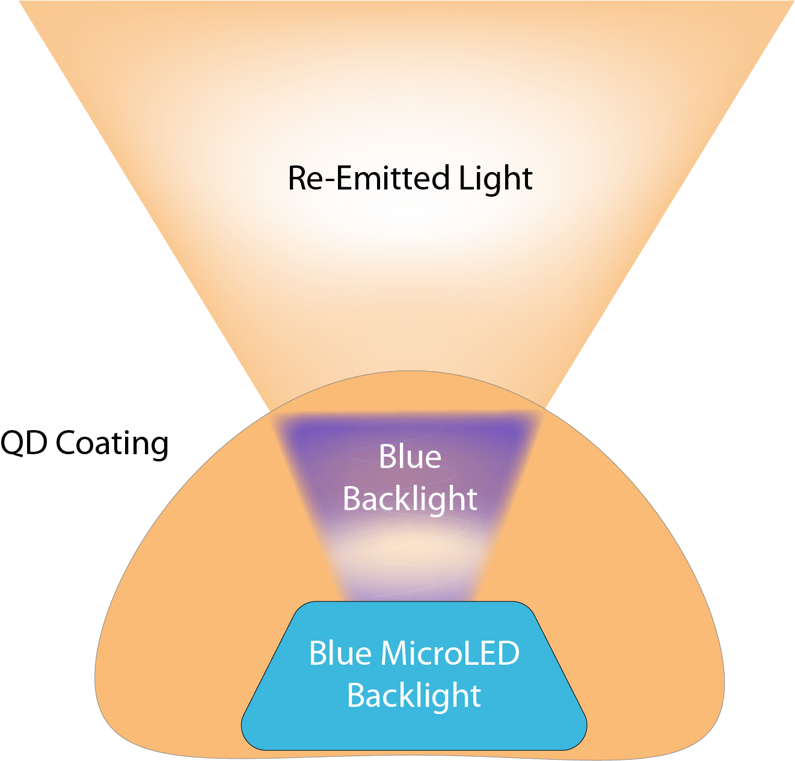
Quantum dots were used to modify the light emissions from the individual micro LEDs
Prospects for Implantable Sensors
Efforts to advance the field of neural imaging with implantable sensors have produced hope for better monitoring of neurological conditions and higher success rates for invasive surgical treatments. In addition to the optical sensors discussed in this article, scientists have made progress in miniaturizing ECoG sensors using organic semiconductors among other new technologies. Coupling progress to improve the sensing hardware with recent achievements in machine learning may provide a pathway to the next generation of clinical care and treatment of neurological diseases.
Resources
To read more about the OLED-based implantable sensor, click here.
To read more about the micro LED-based implantable sensor, click here.
References
Cox, Marshall P., et al. “LED-based optical device for chronic in vivo cerebral blood volume measurement.” IEEE transactions on electron devices 57.1 (2010): 174-177.
Kim, Youngwan, et al. “An ultra thin implantable system for cerebral blood volume monitoring using flexible OLED and OPD.” Electron Devices Meeting (IEDM), 2015 IEEE International. IEEE, 2015.
Choi, Christopher, et al. “Localizing Seizure Activity in the Brain Using Implantable Micro‐LEDs with Quantum Dot Downconversion.” Advanced Materials Technologies (2018): 1700366.
Khodagholy, Dion, et al. “NeuroGrid: recording action potentials from the surface of the brain.” Nature neuroscience 18.2 (2015): 310.