Overview
Individual component materials affect much of the behavior of optical systems. Metamaterial components are no different. While silica-based glass lenses, prisms, and beamsplitters might be the default for many applications, and appropriately so, one of the more interesting subcategories of optical component materials is most often made up of metals and not glass. Today we are exploring this subcategory—metamaterials. A material’s refractive index and its response to an electromagnetic field are two of many parameters considered when designing a system, and metamaterials are very unique in this regard.
So…What Even Are Metamaterials?
The word may be unfamiliar, but its structure hints at the essence of what metamaterials are. Some of the accepted meanings of the prefix ‘meta-’ include ‘beyond’ and even ‘transcending’. Regardless of the definition you are partial to, both are fitting since metamaterials exhibit properties that transcend those inherent to ordinary materials. Metamaterials do not occur naturally; they are crafted to have properties that differ from the properties of the materials, typically metals, that make them up. Such metals include silver and titanium dioxide though researchers at Duke have fabricated a metal-free metamaterial from boron-doped silicon. The component materials, irrespective if they are metallic, are smaller than the wavelength the metamaterial component will be subjected to. The metamaterial’s structural arrangement and size causes it to exhibit desired electromagnetic properties when exposed to an external field. Characteristically, the material structure produces electric and magnetic moments when interacting with electromagnetic waves. These moments then affect the effective permittivity and permeability of medium as a whole. There are different classes of metamaterials based on the material’s signs of the permittivity and permeability, though often metamaterials have both a negative permittivity and permeability resulting in a negative refractive index. Metamaterial components can be used in various applications, a few of which are explored below.
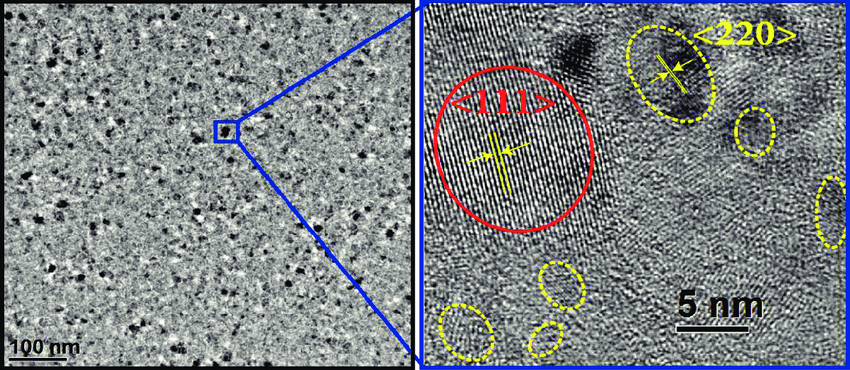
Pictured is a non-metal metamaterial. Each of the lego-like cylinders is made of the boron-doped silicon to create a metamaterial. Note the scale in the lower right hand corner. Courtesy of ResearchGate.
Metamaterial Cloaking
Previously, we have delved into optical cloaking with lenses and temporal cloaking. The article mentioned that there were several other types of cloaking in development. Metamaterial cloaking is one such type. There are subgroups within metamaterial cloaking one of which is the so-called carpet cloaking. Instead of choosing lenses with mismatched focal lengths to cause the light to bypass certain regions, as was the case with refraction-dependent cloak reported by the University of Rochester, the optics themselves bend the light through the employment of transformation optics.
Carpet Cloaking
Carpet cloaking has been found to be successful at different wavelengths, but most notably in the visible range. Unfortunately, carpet cloaks tend to be polarization dependent and unique to the hidden object size. A visualization of how a carpet cloak works is to think of a rug. A rug can be pulled over an object on the floor to conceal it. Likewise, with carpet cloaking an object will be hidden under a metamaterial layer. The carpet cloak itself is seen, but an observer would be unable to discern if an object was beneath it. The metamaterial layer directs the light as to conceal the bump of whatever is hidden under it, so the carpet cloak itself appears smooth.

The left portion of the image displays how an object would be hidden behind the metamaterial layer. The right portion shows how an observer would view the system. Courtesy of Sun and Litchinister
More Cloaking With Metamaterials
One of the other methods of metamaterial cloaking utilizes “anisotropic dielectric permittivity and magnetic permeability” as described by Sun and Litchinister in Fundamentals and Applications of Nanophotonics. When the metamaterial optics are exposed to an electromagnetic field, they distort the incoming wavefront and its phase. This forms a region of space that will cause whatever is behind it to be unseen when viewing.

This displays how the metamaterial bends the light leaving an obscured region in the center area of the cloak. Courtesy of Duke University
As one could imagine, metamaterial components can have limited usage since their electromagnetic responses are wavelength specific. More recently, however, there have been successes with broader ranges of wavelengths and lower losses. Three-dimensional cloaking remains an unsolved problem but has been relatively successful in two dimensions in the instances described above.
The role metamaterials can play in optical cloaking with their uncommon electromagnetic properties is only one of many metamaterial applications.
Superlenses from Metamaterials
The best optical lens-based imaging systems, assuming the absence of all aberrations, are purely diffraction-limited. It has long been wondered if physics could be fiddled with so the resolution could extend beyond that dictated by the airy disk diameter. It was thought this could be achieved using negative refractive index materials. This is why metamaterials have proposed usage in the aptly named ‘superlens’. A negative index is possible through the material’s enhancement and redirection of evanescent waves, whereas normally all that evanescent waves would usually contribute to would be towards the system’s loss.
A group out of Nanyang Technological University in Singapore took a different approach in developing an experimental metamaterial far-field superlens that “beats” the diffraction limit without enhancing the evanescent waves but by taking advantage of optical super-oscillations. The material causes the diffracted waves to interfere at specific amplitude and phase profiles creating a focus smaller than the diffraction limit at the focal plane. This displays the versatility metamaterials can provide—two very different methods for building superlenses, but both can provide superior resolution than was previously accepted.
Because of the improvements on resolution, some have described superlenses as perfect. Although the image resolution can be improved using metamaterials, perfect is a bit of an overstatement. There have been some issues producing sharp images especially in the infrared range with thermal imaging due to the typically metallic, and thus conductive, nature of metamaterials. Therefore, the aforementioned non-metal metamaterial made of born-doped silicon could be of great value since the conductivity of silicon is low.
Conclusion
Metamaterial open the door for all kinds of interesting applications beyond the ones mentioned here. As advancements proceed, it could be expected to see a variety of materials being used for metamaterials beyond metals. Increasing the breadth of allowable wavelengths to increase component usage and to reduce loss would also be anticipated.
This post was sponsored by DataRay Inc. – world leader in laser beam profiling solutions