In the realm of optical engineering and photonics, optical bandpass filters stand as essential yet often underappreciated components. These filters, integral in managing light wavelengths, are crucial in a vast array of applications – from enhancing astronomical observations to advancing biomedical imaging technologies.
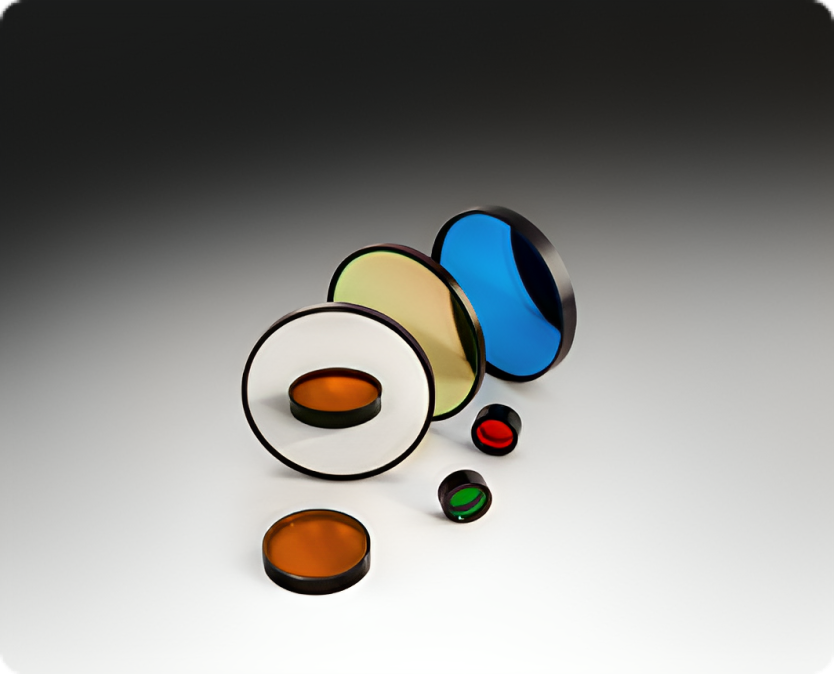
A selection of optical bandpass filters, as discussed in our detailed guide. Image courtesy of Andover Corporation.
This article, “Bandpass Filters Explained: A Detailed Guide,” is designed to unfold the layers of complexity surrounding optical bandpass filters. Aimed at engineers, scientists, and technical enthusiasts, it delves into the principles, types, applications, and nuances of selecting the right optical bandpass filters. Whether you are a seasoned expert or a curious newcomer in the field of optical technology, this guide aims to illuminate the critical aspects of these filters, enhancing your understanding and aiding in informed decision-making for your optical projects.
This guide is brought to you by Zolix Instruments - Empowering Precision Optics with Expert Solutions.
Basic Principles of Optical Bandpass Filters
Optical bandpass filters are specialized components in the field of photonics, designed to transmit light within a specific wavelength range while blocking light outside this range. These filters are pivotal in applications where precise wavelength selection is critical, such as in spectroscopy, laser line separation, or fluorescence microscopy.
At their core, optical bandpass filters operate on the principle of wavelength selectivity. This is achieved through various methods, such as interference, absorption, or a combination of both. Interference filters, for instance, utilize multiple thin layers of different materials to create constructive and destructive interference patterns, allowing only certain wavelengths to pass through. Absorptive filters, on the other hand, rely on materials that inherently absorb specific wavelengths while transmitting others.
What sets optical bandpass filters apart from other types of filters, like longpass or shortpass filters, is their ability to isolate a band of wavelengths. Longpass filters only block wavelengths shorter than a certain threshold, and shortpass filters do the opposite. However, an optical bandpass filter provides a ‘window’ of transmitted light, defined by both an upper and a lower cutoff wavelength. This characteristic makes them exceptionally useful in systems where both the rejection of unwanted light and the passage of a specific spectral region are necessary.
Furthermore, the precision of an optical bandpass filter is defined by its bandwidth, which can vary from very narrow (few nanometers) for high-precision applications to relatively broad for less critical applications. The steepness of the filter’s transition from blocking to transmitting (known as the edge steepness or transition width) also plays a vital role in its performance, especially in applications requiring high spectral resolution.
Types of Optical Bandpass Filters
Optical bandpass filters are pivotal in numerous applications, owing to their ability to selectively transmit specific wavelength ranges. Broadly categorized into interference, absorptive, and dichroic filters, each type comes with its unique set of characteristics, advantages, and ideal applications.
Interference Filters:
Description: These filters use multiple thin-film layers to create constructive and destructive interference, allowing only certain wavelengths to pass through. They are known for their high precision and narrow bandwidths.
Advantages: Interference filters offer excellent wavelength selectivity and high transmission efficiency within their passband. They are highly customizable in terms of bandwidth and center wavelength.
Disadvantages: They can be sensitive to angle and temperature variations, which might alter their spectral characteristics.
Applications: Widely used in spectroscopy, astronomy (for isolating specific spectral lines), and laser-based applications (for filtering specific laser wavelengths).
Absorptive Filters:
Description: These filters rely on the intrinsic properties of materials to absorb unwanted wavelengths while transmitting the desired range. They are often made from colored glass or dyed plastics.
Advantages: Absorptive filters are generally more robust and less sensitive to angle and temperature changes compared to interference filters.
Disadvantages: They usually have broader bandwidths and less precise wavelength control, with lower peak transmission levels.
Applications: Common in photography (for color balancing), basic scientific instruments, and educational tools where extreme precision is not critical.
Dichroic Filters:
Description: Dichroic filters are a type of interference filter that reflects unwanted wavelengths while transmitting the desired range. They are constructed with multiple thin layers of dielectric materials.
Advantages: These filters have high durability and excellent resistance to heat and humidity. They offer sharp cutoffs between transmitted and reflected wavelengths.
Disadvantages: Like other interference filters, they can be sensitive to the angle of incidence of the light.
Applications: Often used in fluorescence microscopy (for directing specific wavelengths to the detector), RGB color mixing in projectors, and advanced lighting systems.
Each type of optical bandpass filter serves a unique purpose in the world of optics. The choice of filter depends on the specific requirements of the application, such as the precision of wavelength selection, environmental tolerance, and the intensity of light that needs to be managed. Understanding the strengths and limitations of each filter type empowers engineers and scientists to make informed decisions for their optical systems.
Key Specifications and Performance Parameters
Understanding the key specifications and performance parameters of optical bandpass filters is essential for selecting the right filter for specific applications. The primary specifications include bandwidth, center wavelength, and transmission levels, each playing a crucial role in determining the filter’s performance.
1. Bandwidth of Bandpass Filters:
- Explanation: Bandwidth refers to the range of wavelengths that the filter allows to pass through. It’s typically defined as the difference between the upper and lower cutoff wavelengths where the transmission falls to a specific percentage of the peak transmission (often 50%).
- Performance Impact: A narrower bandwidth offers more precise control over the transmitted wavelengths, which is vital in applications like spectroscopy or laser line isolation. Conversely, a broader bandwidth is suitable for applications where such precision is less critical.
- Application Relation: In fluorescence microscopy, narrow bandwidths help in isolating specific fluorescence signals, whereas in general lighting applications, broader bandwidths are often sufficient.
2. Center Wavelength:
- Explanation: The center wavelength is the midpoint of the bandpass range and is where the filter typically has its peak transmission.
- Performance Impact: The accuracy of the center wavelength is crucial in applications where specific wavelength targeting is necessary. Any deviation can lead to incorrect or inefficient filtering.
- Application Relation: In astronomical imaging, precision in the center wavelength is essential to capture specific astronomical phenomena, while in general color filtering applications, slight variations might be tolerable.
3. Transmission Levels:
- Explanation: Transmission levels indicate the percentage of light transmitted through the filter at its peak. High transmission levels mean more light passes through at the desired wavelength.
- Performance Impact: Filters with higher transmission levels are more efficient, providing brighter images and better signal-to-noise ratios. However, they may also transmit more of the unwanted light if not precisely designed.
- Application Relation: High transmission levels are particularly important in low-light applications, such as in astronomy or biomedical imaging, where maximizing signal capture is crucial.
Each of these parameters must be carefully considered in relation to the intended application. For instance, in high-precision scientific instruments, narrow bandwidths, precise center wavelengths, and high transmission levels are typically necessary for accurate and efficient performance. In contrast, for broader commercial applications, there may be more flexibility in these specifications. Ultimately, the optimal balance of these parameters depends on the specific requirements of the application, including considerations of the light source, the sensitivity of detection equipment, and the nature of the target signal or image.
Design and Fabrication Considerations
Design and Fabrication Considerations for Optical Bandpass Filters
The design and fabrication of optical bandpass filters involve a meticulous selection of materials, layer design, and coating technologies. These factors are critical in determining the filter’s performance, durability, and suitability for specific applications.
Filter Materials: The choice of material is paramount in filter design. Materials like fused silica, borosilicate glass, and various types of crystals are commonly used. Each material has distinct optical properties, like transmission ranges and thermal stability, influencing the filter’s performance. For instance, fused silica is preferred for its high transmission in the ultraviolet (UV) range, making it suitable for UV applications.
Layer Design: Optical bandpass filters typically comprise multiple thin layers of different materials. These layers create the required interference patterns to select specific wavelengths. The precision in the thickness and uniformity of these layers is crucial. Variations can significantly impact the filter’s bandwidth and center wavelength. Advanced techniques like ion-beam sputtering and vacuum deposition are employed to achieve the necessary precision.
Coating Technologies: Coating technologies play a vital role in defining the filter’s characteristics. Techniques like dielectric coating, where multiple layers of non-conductive materials are deposited, are widely used. These coatings must be stable, durable, and resistant to environmental factors like humidity and temperature changes.
Challenges in Fabrication:
One of the primary challenges in fabricating optical bandpass filters is maintaining the precision of the layer thickness and material quality across the entire filter surface. Any inconsistency can lead to variations in filter performance.
Another challenge is ensuring the filter’s longevity and resistance to environmental factors, which requires robust coating technologies and stringent quality control during the fabrication process.
Additionally, as the demand for narrower bandwidths and higher transmission efficiency increases, particularly in sophisticated applications like telecommunications and medical imaging, the fabrication process becomes increasingly complex, requiring cutting-edge technology and expertise.
In conclusion, the design and fabrication of optical bandpass filters require a careful balance of material properties, layer precision, and advanced coating techniques. These factors must be meticulously managed to produce filters that meet the stringent demands of diverse optical applications.
Applications of Optical Bandpass Filters
Optical bandpass filters have revolutionized various fields by their ability to precisely control the wavelengths of light passing through them. Their applications span from astronomy to biomedical imaging, each utilizing the unique properties of these filters.
Astronomy: In astronomy, optical bandpass filters are indispensable for observing celestial bodies and phenomena. They enable astronomers to isolate specific wavelengths emitted by stars, planets, and galaxies, providing insights into their composition and behavior. For instance, a hydrogen-alpha filter is often used to observe solar flares and prominences on the sun, allowing for detailed study of solar activity.
Photography: Photographers use optical bandpass filters to enhance image quality and achieve artistic effects. Infrared filters, for example, are popular for creating surreal landscapes where foliage appears white and skies dark, highlighting contrasts not visible to the naked eye. Such filters are instrumental in both artistic and scientific photography, including aerial and environmental surveying.
Biomedical Imaging: In the biomedical field, these filters are critical in fluorescence microscopy. They allow for the observation of specific cellular components tagged with fluorescent markers, aiding in the diagnosis and research of diseases. By isolating the wavelengths emitted by these markers, researchers can obtain clear, precise images of cellular structures and processes.
Laser Systems: Optical bandpass filters are also key components in laser systems, particularly in laser-based measurement and communication technologies. They ensure the purity of the laser light by filtering out unwanted spectral noise, thus enhancing the system’s accuracy and efficiency.
A Case Study for Optical Bandpass Filters
A notable example is the use of optical bandpass filters in the Hubble Space Telescope. The telescope is equipped with a variety of filters that allow astronomers to observe different wavelengths emitted by distant celestial objects. This capability has been pivotal in making groundbreaking discoveries about the universe, including the rate of its expansion and the properties of distant galaxies.
In summary, optical bandpass filters are versatile tools that significantly enhance the capabilities of various technologies in astronomy, photography, biomedical imaging, and laser systems. Their ability to selectively transmit specific wavelengths of light makes them invaluable in both scientific research and practical applications.
Buying Guide for Optical Bandpass Filters
When purchasing optical bandpass filters, several key factors must be considered to ensure that the filter meets the specific requirements of your application. Understanding these factors can help in selecting the right type of filter while ensuring quality and durability.
Optical Specifications:
The most critical aspect to consider is the filter’s optical specifications, which include bandwidth, center wavelength, and transmission levels. Ensure these align with your application’s requirements. For high-precision applications, like in scientific research, look for filters with narrow bandwidths and accurate center wavelengths. For broader applications, such as in general photography, slightly less precise specifications may be acceptable.
Filter Quality:
The quality of the filter is paramount. This includes the uniformity of the coating, the quality of the substrate, and the overall fabrication precision. High-quality filters offer better performance, more accurate results, and reduced likelihood of introducing artifacts or errors into your system.
Manufacturer Reputation and Reliability:
Research the manufacturer’s reputation in the market. Established manufacturers with a history of producing high-quality optical filters are generally more reliable. Look for reviews, testimonials, and case studies that demonstrate their expertise and product performance.
Compatibility with Existing Systems:
Ensure the filter is compatible with your existing optical system, including size, mounting requirements, and spectral compatibility. This is crucial for integrating the filter seamlessly into your setup without needing additional modifications.
Application-Specific Requirements:
Different applications may have unique requirements. For instance, filters used in harsh environmental conditions, such as in outdoor photography or industrial settings, should have robust environmental resistance. In contrast, filters used in controlled environments, like laboratories, can focus more on spectral precision.
Maintenance and Durability:
Consider the maintenance needs and durability of the filter. A filter that is easy to clean and resistant to scratches and other damage can be a more cost-effective choice in the long run. Check for any special handling or storage requirements to maintain the filter’s performance over time.
Cost vs. Performance:
Balance the cost against the performance benefits. While higher-quality filters may come at a premium, they often provide better performance and longevity, which is often more cost-effective in the long run, especially for critical applications.
In summary, when buying an optical bandpass filter, it’s important to thoroughly evaluate your specific application needs, the quality and specifications of the filter, the manufacturer’s reputation, and the overall cost-effectiveness. Making an informed decision based on these factors will ensure that you select a filter that meets your requirements and provides reliable performance.
Conclusion
Optical bandpass filters are integral components in a wide range of scientific and technical applications, from astronomy and photography to biomedical imaging and laser systems. Understanding their types, from interference to absorptive and dichroic filters, and their respective advantages, is crucial for their effective utilization. Key specifications like bandwidth, center wavelength, and transmission levels play a pivotal role in their performance and suitability for specific tasks.
When selecting an optical bandpass filter, it’s essential to consider factors such as optical specifications, quality, manufacturer reputation, and compatibility with existing systems. The right choice not only enhances the efficiency and accuracy of your application but also contributes to the longevity and reliability of your optical system. Therefore, investing time in understanding these filters and carefully selecting the appropriate one for your needs is imperative for achieving optimal results in any field where precise light manipulation is required.
Further Reading on Optical Bandpass Filters
For those seeking to delve deeper into the world of optical bandpass filters, the following references and sources provide extensive information and insights:
- “Handbook of Optical Filters for Fluorescence Microscopy” by J.R. Lakowicz. A detailed guide focusing on the use of optical filters in fluorescence microscopy, discussing various types and their applications.
- “Advanced Photonics with Second-order Optically Nonlinear Processes” by A.D. Boardman et al. This research paper delves into advanced optical processes, including the role of bandpass filters in nonlinear optics.
- “Fundamentals of Optical Waveguides” by Katsunari Okamoto. This book provides an in-depth understanding of optical waveguides. It also includes information on the use of optical filters in these systems.
- “Spectral Imaging: Principles and Applications” in the journal “Biomedical Spectroscopy and Imaging”. This journal article discusses spectral imaging techniques and the role of optical bandpass filters in these applications.
- “Design of Thin Film Coating Systems for Bandpass Filters” in the journal “Thin Solid Films”. This paper offers insights into the design considerations and fabrication techniques for thin-film bandpass filters.
- NASA’s Technical Reports Server (NTRS): A repository of papers and reports on various space-related technologies, including the use of optical bandpass filters in space telescopes and other astronomical instruments.
- “The Physics and Engineering of Solid State Lasers” by Yehoshua Kalisky. This text covers the engineering aspects of solid-state lasers, including the application of optical bandpass filters.
Each of these sources provides a unique perspective and level of detail on optical bandpass filters, making them invaluable for anyone looking to enhance their understanding or research in this field. Whether you’re a student, a researcher, or a professional, these references will serve as a solid foundation for further exploration and study.