In optical spectroscopy, the quantification of light absorption as it transmits through a medium is foundational to many analytical techniques. Beer-Lambert’s Law, a linear relationship that correlates the absorbance of light with the properties of the material through which it passes, serves as a critical tool for scientists and engineers. This law enables precise measurements of concentration and purity in substances by analyzing how they absorb light at specific wavelengths. As we look into the principles and applications of Beer-Lambert’s Law, we uncover its role in advancing science and practical application across disciplines ranging from biochemistry to industrial manufacturing.
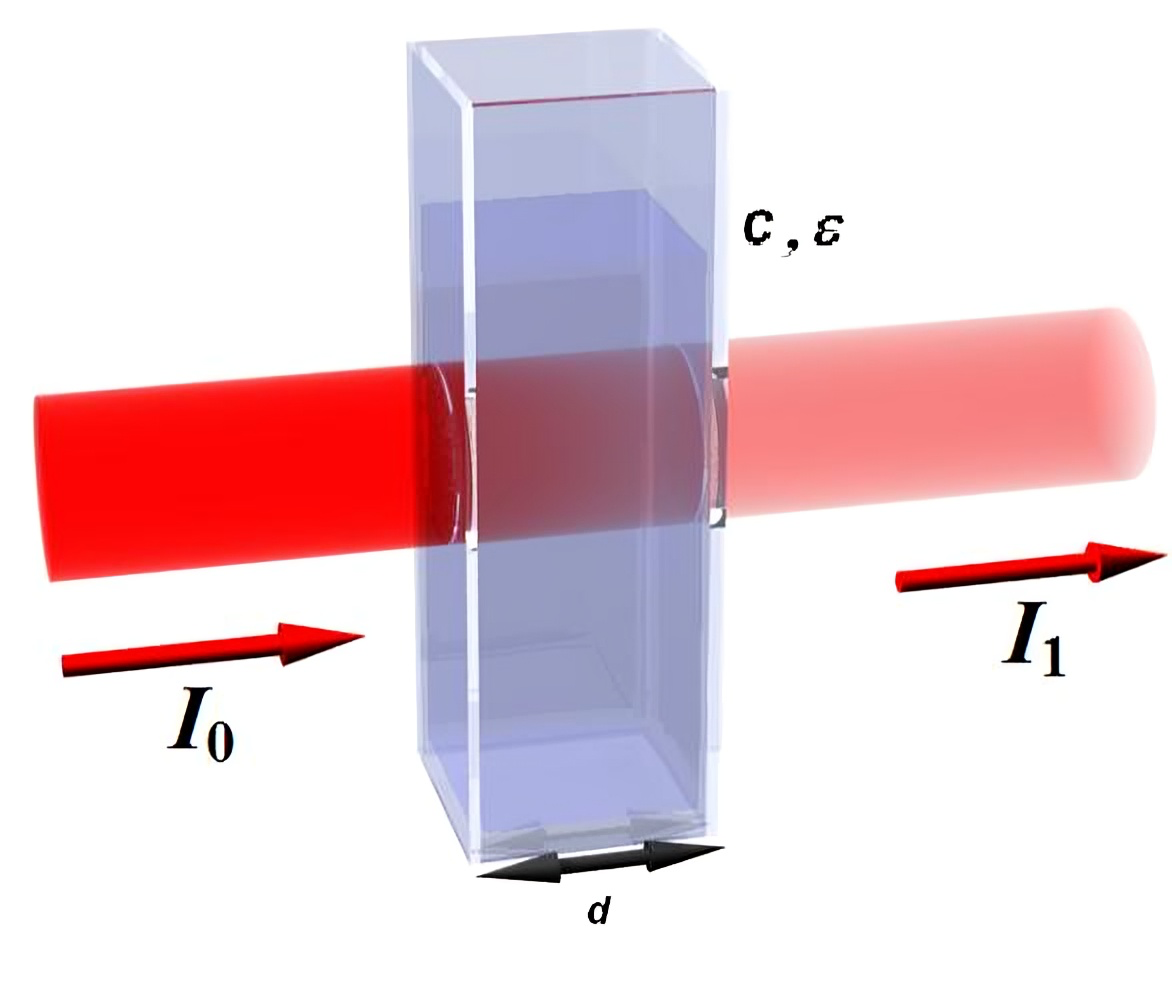
Beer-Lambert’s Law defines the relationship between the absorbance of light by a substance and its concentration, expressing that absorbance is directly proportional to both the path length of light through the medium and the concentration of the absorbing species. Image Courtesy of Wikimedia.
1. Introduction
Beer-Lambert’s Law, a fundamental principle in spectroscopy, describes how the intensity of light absorbed by a medium correlates with the properties of that medium. Specifically, it quantifies the absorption as proportional to the concentration of the substance and the path length the light travels through it. This linear relationship is pivotal in fields such as chemistry, where it underpins analytical methods to determine the concentration of solutes in a solution. In biology, the law is crucial for studying biochemical pathways by analyzing light absorption patterns of different biomolecules. Moreover, environmental scientists rely on this law to monitor pollutants by measuring the absorbance of light through atmospheric or water samples. Understanding Beer-Lambert’s Law thus provides essential insights into material properties and aids in the development of technologies and solutions across a broad spectrum of scientific endeavors.
2. The Basics of Beer-Lambert’s Law
At its core, Beer-Lambert’s Law provides a mathematical model to describe the attenuation of light as it passes through an absorbing medium. The law is expressed by the equation:
A
where:
- is the absorbance, which is a dimensionless quantity and indicates how much light is absorbed by the sample.
- (epsilon) is the molar absorptivity or molar extinction coefficient, which characterizes how strongly a chemical species absorbs light at a given wavelength and has units of Lâ molâ1â cmâ1.
- c is the concentration of the absorbing species within the medium, measured in moles per liter (mol/L).
- l is the path length that light travels through the sample, typically in centimeters.
Understanding the Components:
- Absorbance (A): It is the logarithmic measure of the ratio of incident light to transmitted light through a substance. It indicates the amount of light absorbed by the medium and is a key output in spectrophotometry experiments.
- Path Length (l): This refers to the distance that light travels through the absorbing medium. In most spectrophotometric studies, this is the width of the cuvette containing the sample, which is typically standardized at 1 cm.
- Concentration (c): The amount of the substance dissolved in a given volume of solvent, which directly affects how much light is absorbed, provided the path length and molar absorptivity are constant.
- Molar Absorptivity (Δ): A constant that indicates the absorbance of light per unit concentration and path length for a particular substance at a specific wavelength. This value is inherent to each substance and crucial for calculating concentrations in unknown samples.
Beer-Lambertâs Law allows for the precise quantitative analysis of a substanceâs concentration in a solution by measuring how much light it absorbs, thereby serving as the cornerstone of many analytical techniques in scientific research.
3. How Beer-Lambert’s Law Works
To understand how Beer-Lambert’s Law applies in practice, let’s explore the step-by-step process of light absorption and transmission through a medium. This explanation will highlight the relationship between light intensity, concentration of the substance, and the path light travels.
- Incident Light: Light of a specific wavelength is directed towards a sample. The choice of wavelength generally corresponds to the maximum absorbance wavelength of the substance being analyzed.
- Interaction with the Medium: As the light enters the sample solution in a cuvette, it encounters molecules of the absorbing species. Depending on the properties of these molecules, a certain amount of light is absorbed.
- Transmission of Light: The light that is not absorbed continues to travel through the solution. This transmitted light reaches the detector on the other side of the cuvette.
- Measuring Absorbance: A spectrophotometer measures the intensity of incident light (Iâ) and the intensity of transmitted light (I). Absorbance (A) is then calculated using the logarithmic relationship: A=âlogâĄ10(I/I0)This equation reflects how much light is absorbed as it passes through the sample.
- Relating Absorbance to Concentration and Path Length: From Beer-Lambertâs Law (A=Ï”â câ l), absorbance is directly proportional to the concentration of the absorbing species in the solution and the path length of the cuvette. Increasing either the concentration or the path length will result in higher absorbance, assuming the molar absorptivity remains constant.
By correlating the detected absorbance with known values of Δ and l, scientists can determine the concentration of the substance in the sample. This process exemplifies how integral Beer-Lambert’s Law is to quantitative analytical methods in scientific research.
4. Applications of Beer-Lambert’s Law
Beer-Lambert’s Law is not only a fundamental scientific concept but also a practical tool used in various industries and fields of study. Below are key areas where this law plays a crucial role:
Medical Applications:
- Blood Oxygen Levels: Did you know, that pulse oximeters, devices that estimate the oxygen saturation of a patient’s blood, operate on principles derived from Beer-Lambert’s Law. By analyzing the absorption of light through a patient’s fingertip or earlobe at different wavelengths, these devices can discern the proportion of oxygenated and deoxygenated hemoglobin.
- Other Bodily Fluids: The law is also applied in clinical diagnostics to measure the concentrations of various substances in bodily fluids, such as bilirubin in blood, which is critical for diagnosing liver functions and other medical conditions.
Environmental Science:
- Measuring Pollutants: Environmental monitoring often relies on spectroscopic methods based on Beer-Lambert’s Law to determine the concentration of pollutants in water and air. For instance, the law helps quantify the levels of harmful chemicals like benzene in drinking water or mercury in the air, aiding in compliance with environmental regulations and public health safety.
- Atmospheric Studies: The law is crucial in remote sensing techniques used to analyze atmospheric gases, particularly in studying ozone layers and greenhouse gas concentrations.
Industrial Applications:
- Food and Beverage Quality Control: In the food industry, spectrophotometry is used extensively to ensure the quality and safety of products. For example, Beer-Lambert’s Law is applied to measure the concentration of food dyes in drinks and confectioneries, ensuring they are within safe consumption limits.
- Chemical Analysis: The law also facilitates the monitoring and control of concentrations in chemical manufacturing processes, crucial for product consistency and compliance with industry standards.
Bridging Theory and Practice: These applications demonstrate how Beer-Lambert’s Law bridges theoretical physics and practical technology, significantly impacting daily life and industrial operations. By providing a means to measure concentrations accurately, this law enhances our capability to monitor, diagnose, and manufacture in a way that is safe, efficient, and environmentally responsible.
5. Challenges and Limitations
While Beer-Lambert’s Law is invaluable for quantitative analysis in many scientific fields, it has limitations that must be considered to ensure accurate results:
Deviations at High Concentrations: Beer-Lambert’s Law assumes that absorbance is linearly related to concentration, which holds true under ideal conditions. However, at high concentrations, the interactions between absorbing molecules can lead to deviations. These interactions, such as dimerization or aggregation, can cause the absorbance to no longer be directly proportional to concentration. To address this, professionals often dilute the sample to concentrations where the law remains applicable. Furthermore, they may employ mathematical models to correct for the non-linearity or use alternative spectroscopic methods that are less sensitive to concentration effects.
Chemical Composition and Interaction Effects: The effectiveness of Beer-Lambert’s Law can also be compromised by the chemical composition of the medium. Variations in pH, the presence of other reactive species, or changes in solvent properties can affect the molar absorptivity of the analyte. Professionals address these challenges by rigorously controlling experimental conditions and using buffers to stabilize pH levels. They also ensure that the solvent’s properties are well understood and consistent throughout experiments.
Path Length Variability and Instrumental Errors: The accuracy of measurements under Beer-Lambert’s Law is also dependent on the precision with which the path length and the intensity of light are controlled and measured. Inaccuracies in path length or fluctuations in light source intensity can lead to significant errors in absorbance measurements. To overcome these limitations, high-quality, well-calibrated spectrophotometric equipment is used. Calibration routines are regularly performed to ensure that the path length and light intensity measurements are accurate and reliable.
6. Advanced Concepts and Recent Research
Beer-Lambert’s Law continues to be a foundational principle in spectroscopy and analytical chemistry, but modern research has pushed its boundaries and adapted its core principles to new contexts and technologies:
Adaptations in Non-Linear Spectroscopy: Researchers are exploring the extension of Beer-Lambert’s Law into non-linear optical phenomena where traditional linear assumptions do not hold. In non-linear spectroscopy, the absorbance can depend on the light intensity, leading to applications that investigate the dynamic behaviors of materials under high-intensity light. This research is crucial for developing new materials for lasers and other optical devices.
Multi-Component Analysis: Advancements have also been made in the simultaneous analysis of multiple components in a mixture using modified versions of Beer-Lambert’s Law. Techniques such as derivative spectroscopy and multi-wavelength analysis allow researchers to deconvolute overlapping absorption peaks, enabling more precise quantification of complex mixtures in pharmaceuticals and environmental samples.
Integration with Machine Learning: Recent studies have integrated Beer-Lambert’s Law with machine learning algorithms to enhance the predictive accuracy of spectroscopic analysis. These approaches use large datasets to model the non-linearities and interactions that traditional Beer-Lambert applications might overlook, improving diagnostics in medical imaging and environmental monitoring.
Microfluidic Applications: In the field of microfluidics, researchers have miniaturized spectrophotometric systems that utilize Beer-Lambert’s Law for on-chip chemical analysis. These systems benefit from the lawâs simplicity and are being used in portable devices for on-site testing in fields ranging from medical diagnostics to environmental science.
These innovations demonstrate the adaptability of Beer-Lambert’s Law to modern scientific challenges, highlighting its ongoing relevance and utility in cutting-edge research. Each adaptation not only extends the law’s applications but also contributes to a deeper understanding of light-matter interactions under varying conditions.
7. Conclusion
Beer-Lambert’s Law, with its robust framework for understanding light absorption, continues to be an important concept in the fields of chemistry, biology, and physics. By quantifying how light interacts with materials, this law enables precise measurements crucial for a myriad of applications â from medical diagnostics to environmental monitoring and industrial quality control.
As we’ve explored, despite its fundamental nature, Beer-Lambert’s Law is adaptable and continues to evolve. The advancements in non-linear spectroscopy, multi-component analysis, integration with machine learning, and microfluidic technologies underscore its dynamic and versatile nature. These innovations not only broaden the scope of its application but also enhance the accuracy and efficiency of analytical methods.
Continued study and experimentation with Beer-Lambert’s Law equip researchers and professionals with the tools to innovate and solve complex problems in science and industry. Embracing the ongoing research and technological integration will undoubtedly yield new insights and breakthroughs, reinforcing the enduring relevance of this fundamental law in science.
In conclusion, the journey through the depths of Beer-Lambert’s Law is far from complete. Its continued relevance and adaptation in research invite us all to explore and contribute to the fields of optical spectroscopy and analytical chemistry, ensuring that we remain at the cutting edge of scientific exploration.