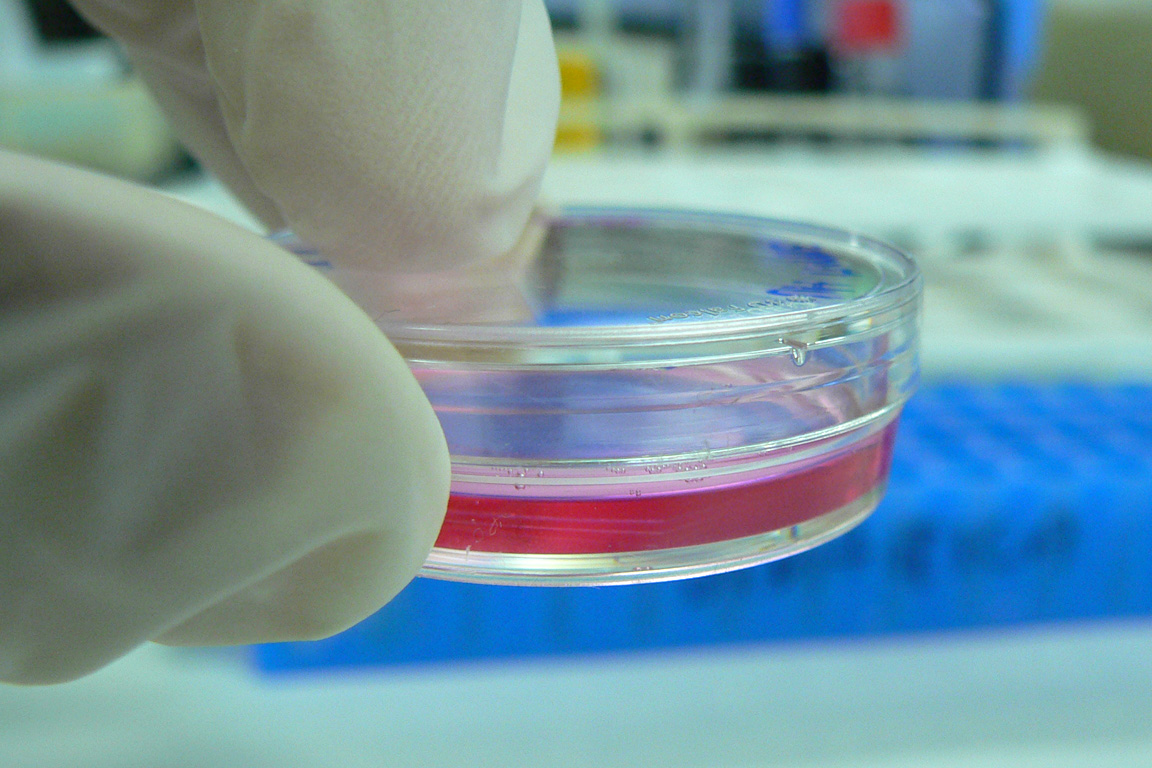
Biodegradable polymers have many uses in the field of tissue engineering. Laser processing offers an advantageous method to manufacture and customize these polymers. Image courtesy of Wikimedia Commons.
A team of researchers from Keio University in Japan has published their results on utilizing laser processing to produce biodegradable polymers for use in tissue engineering. Biocompatible polymers are used extensively throughout tissue engineering. Manufactured scaffolds are vital to the success of engineered tissue. Scaffolds provide support, and oftentimes, nutrition, to the growing tissue. For growing tissue placed inside a body, a second surgery is often required to remove the scaffold after the tissue is self-sustainable. Therefore, biodegradable scaffolds are advantageous as removal is not necessary. Luckily, most material for biodegradable scaffolds are biocompatible regarding implantation in the body, and can be considered for practical medical applications. Another use for such polymers are biodegradable microcapsules, which store drug molecules. Depending on the drug, the microcapsule needs to rupture at different points of time after injection or ingestion. Customization of the microcapsules depends on modifying the degradation rate of materials.
Laser processing has increasingly become more popular for the manufacturing of medical tools. Using lasers reduces the need of employing production techniques used in dry processing while decreasing toxic chemical byproducts. Additionally, the use of lasers increases accuracy and facilitates the customization of the final product. Coincidentally, for implantable medical or surgical devices, the potential for mass customization is incredibly useful. For this reason, the team from Keio University researched the potential for successfully manufacturing biodegradable polymers through laser processing.
Laser Processing of Biodegradable Polymers
Vital to the experiment was the use of the femtosecond laser. Femtosecond lasers are categorized as both ultrafast and ultrashort, in respect to their pulses. These properties allow for a very precise output of the laser, which can easily be programmed for manufacturing three-dimensional structures, on both the surface and on the interior of a polymer. Femtosecond lasers are also ideal for the processing of biodegradable polymers, as laser irradiation of the polymers can induce the scission of chemical bonds. This will, in turn, modify the rate of degradation of the polymer, providing further customization of the part. Finally, the use of a femtosecond laser is advantageous as it produces a minimal heat-affected zone, in comparison to other lasers. This is due to its short interaction time with the material, made possible by its short pulses.
The researchers observed the effects of lasers with 266nm wavelengths, 400nm wavelengths and 800nm wavelengths. The materials used were polylactic-co-glycolic acid (PLGA), which is FDA approved for therapeutic uses, due to its biocompatibility and biodegradation, and poly-L-lactic acid (PLLA). Two shapes were manufactured on the polymers: craters on PLGA films, and laser-induced periodic surface structures on PLLA surfaces. Laser-induced periodic surface structures (LIPSS), also known as “ripples,” are nano-structures produced via linearly polarized irradiation.
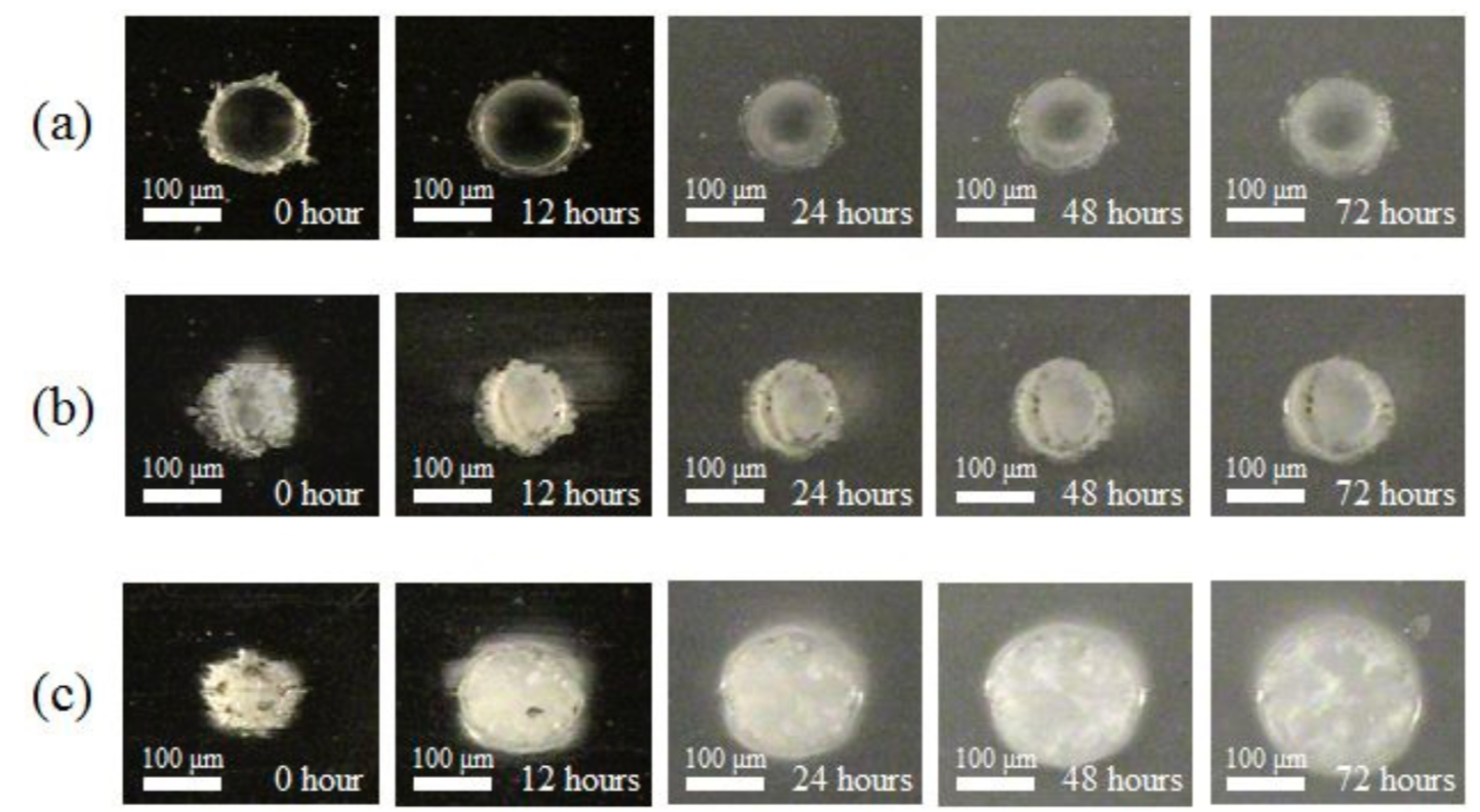
The figure above displays the craters formed on the PLGA forms. The (a) row displays a crater formed by mechanical milling as it degrades over time. The (b) row displays a crater formed by the 800nm laser as it degrades over time. The (c) row displays a crater formed by the 400nm laser as it degrades over time. Image courtesy of MDPI: applied sciences.
Properties of the Manufactured Biodegradable Polymers
Results confirmed that laser wavelength affected degradation rate of the polymer. When examining the craters formed in the PLGA films via mechanical milling, a 400nm femtosecond laser, and an 800nm femtosecond laser, the films treated by the 400nm femtosecond laser degraded significantly faster than the other films. Faster degradation was also observed in the films treated by the 800nm laser compared to the control. This change in degradation rate is explained as surface melting decreases crystallinity of polymer structures and because molecular weight decreases as chemical bonds undergo photolysis. As the samples treated by the 400nm laser degraded more than those treated by the 800nm laser, it was concluded that a significant change in degradation observed by the 400nm samples was due to chemical bond dissociation, and the resulting decrease in molecular weight.
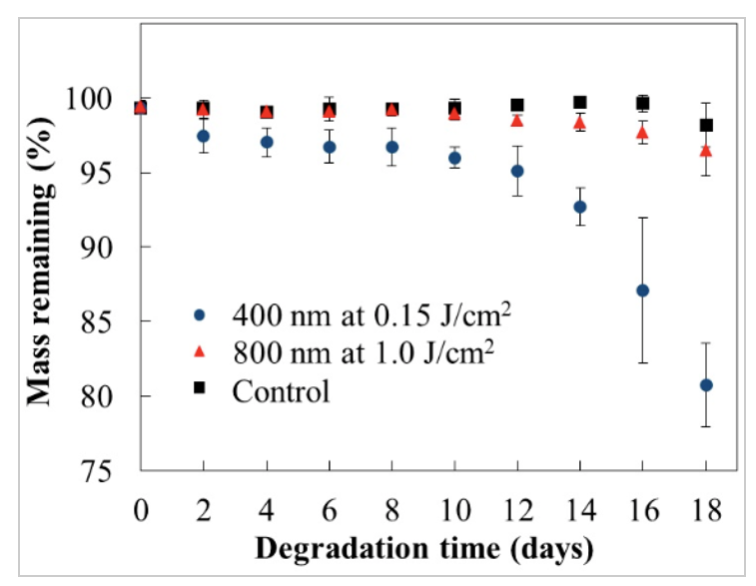
The figure above displays the degradation of the polymer as a function of time. The degradation of three groups of PLGA films: the control group, those treated with a 400nm laser, and those treated with an 800nm laser. Image courtesy of MDPI: Applied Sciences.
After observing the periodic surface structures on the PLLA surface, it was determined that the 400nm wavelength laser was most ideal. Using the 800nm laser rarely resulted in LIPSS, unless perfectly ideal experimental conditions were obtained. This is because the number of photons necessary for multi-photon absorptions is lower at 400nm than at 800nm due to the higher photon energy at 400nm. At 266nm, the structures were very shallow. Researchers hypothesized that the mechanism for structure formation may be different at very low wavelengths, such as 266nm. It is possible that the photomechanical pressure associated with the thermal effect of laser pulses affects LIPSS formation.
Conclusion
The research team confirmed previously published literature that the properties and physical structure of biodegradable polymers can be modified through laser properties. This will improve medical applications of these polymers. By influencing the degradation rate of polymers, the substances can be optimized for molecular drug release in the body. Additionally, modification of this rate allows for a customized lifespan of scaffolds used for engineered tissue. Observing the formation of LIPPS allowed researchers to see the potential of lasers as a powerful tool to modify such biodegradable polymers. Researchers concluded that further studies will allow precise fabricated biodegradable polymers to become reality for the medical field soon. These studies will benefit from new techniques such as cooled laser ablation or the use of a Bessel beam as the field of laser processing progresses.
Laser processing is a very well researched manufacturing technique, and has potential in many fields. Modification and customization of polymers for medical and in vivo use will certainly bring large benefits to the medical field.
For more info, the published paper, which includes the review of relevant literature as well, can be read here.