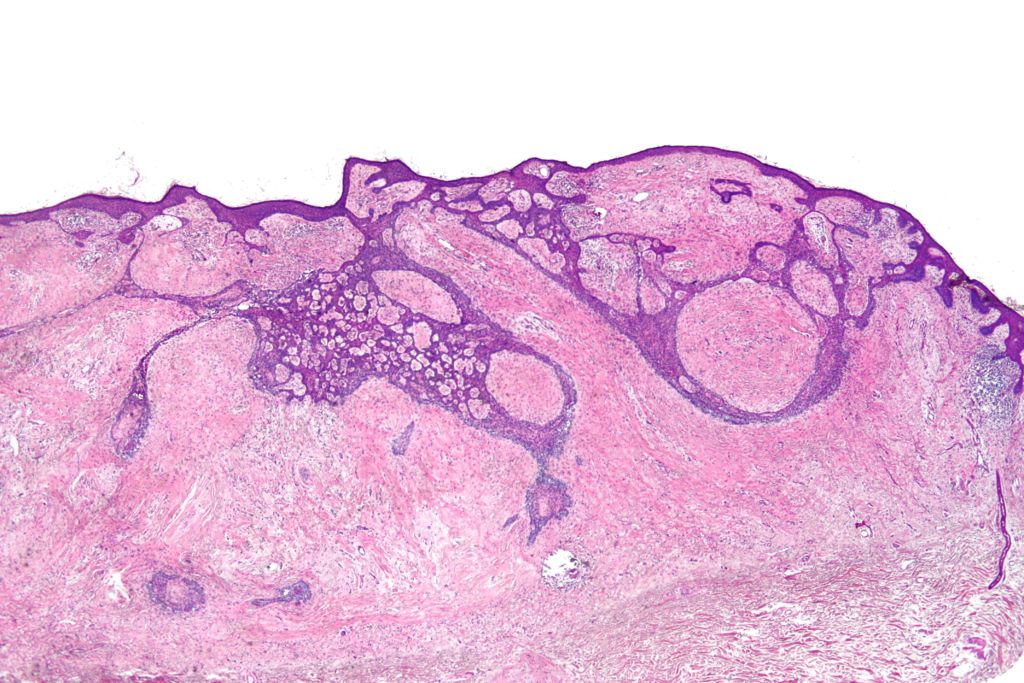
Basal cell carcinomas is one of the most diagnosed forms of cancer worldwide. A biophysical cancer analysis technique using Raman spectrometry would greatly improve success of an individual with basal cell carcinoma following resection surgery. Image courtesy of Wikimedia Commons.
A research team from the University of Texas at Austin has published their findings on using Raman spectrometry for biophysical cancer analysis to analyze skin cancer. Their findings were applied to assist in Mohs micrographic surgery during basal cell carcinoma resections. Raman spectrometry is used to find the biophysical characteristics of a tumor that would identify it as cancerous. This information assists surgeons in determining appropriate resection margins. In turn, this decreases the chances of cancer recurrences and increases success of the surgery.
What is Raman Spectrometry?
Raman spectrometry is a spectroscopic technique which examines molecular vibrations. It uses a laser in the near infrared or near ultraviolet range to irradiate a sample in question. After irradiation, molecules will reflect and scatter light at a different wavelength, a phenomenon referred to as the Raman effect.
Raman spectrometry measures the inelastically scattered light, known as the Raman shifted light, or Stokes. These Stokes are then collected by a filter, which filters out the Rayleigh and Anti Stokes scattering. A diffraction grating then bends the Raman shifted light according to wavelength, and a detector records and passes the signal to a computer to be decoded. The signal is then displayed on a graph known as a Raman spectra, which plots the intensity of the scattered light for each energy of light.
Why use Raman spectrometry for biophysical cancer analysis?
One major factor influencing the Raman scattering intensity measured in Raman spectrometry is the polarization state of molecules being irradiated. If the change in polarizability is large, the intensity will be high. Therefore, as the reflected wavelength is dependent upon the specific chemical composition of each molecule, Raman spectrometry can help identify between various substances. Compared to traditional histology techniques, it has higher resolution and requires minimal tissue processing.
By analyzing skin samples and finding the Raman spectra of various components, a biophysical model of skin can be made, as shown below. From this, relevant biophysical markers can be identified. These markers can then be used to distinguish between areas of normal skin and areas of basal cell carcinomas.
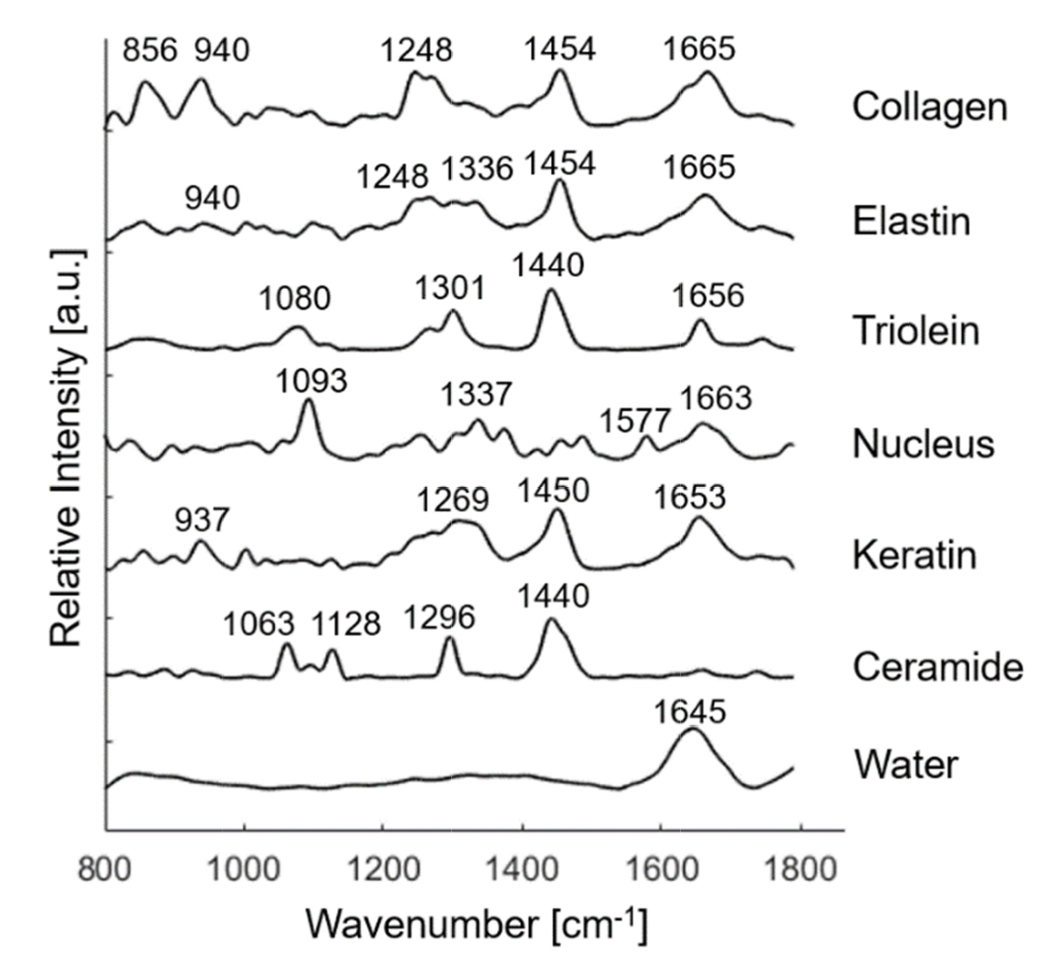
The above graph displays the Raman spectra associated with various Raman active components found in skin tissue structures. Peak positions of the Raman bands are identified and labeled. Image courtesy of Biomedical Optics Express.
Raman spectrometry can then, therefore, be used to assist surgeons in identifying proper resection margins for Mohs micrographic surgery.
The Experiment
During the research into the effectiveness of Raman spectrometry in identifying between normal and cancerous skin tissue, frozen samples from the Austin Dermatologic Surgery Center were examined. Each sample was examined using both Raman spectrometry and traditional histology techniques for comparison. A custom-made confocal Raman spectrometer with a wavelength of 830nm was used. This wavelength was chosen as it would minimize tissue auto-fluorescence. To further remove tissue fluorescence background noise, raw Raman spectra data was filtered using wavenumber calibration, dark noise removal, cosmic ray removal, smoothing, and a fifth-order polynomial fitting.
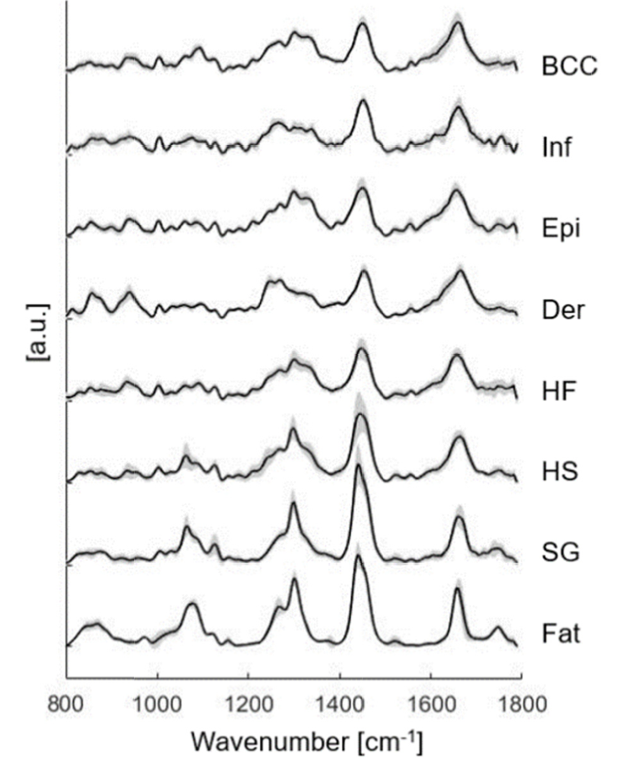
The figure displays various Raman spectra of the many skin structures, including basal cell carcinomas (BCC), inflamed dermis (Inf), epidermis (Epi), dermis (Der), hair follicle (HF), hair shaft (HS), sebaceous gland (SG) and fat. These Raman spectra were based on the mean Raman spectra with standard deviation included for each structure. Image courtesy of Biomedical Optics Express.
Raman spectra of potentially cancerous skin tissue was then compared to the Raman spectra of known skin structure samples, to determine the state of the unknown sample. Additionally, results were compared to histology results to test the sensitivity and specificity of the results obtained through Raman spectrometry.
Conclusion
Following statistical analysis, it was found that Raman spectrometry was 90% sensitive and 92% specific when identifying basal cell carcinomas. The research confirmed that there are significant differences in biochemical and structural compositions between basal cell carcinomas and normal skin. Specifically, the spectra of basal cell carcinomas had larger contributions from nucleus material compared to normal skin. Additionally, keratin contributed more to basal cell carcinomas compared to both inflamed and normal dermis. It has been proven that keratin expression is related to tumor differentiation, and therefore, can provide insight into tumor origins.
The test could be modified to prioritize high specificity or high sensitivity. High specificity is important in surgeries such as Mohs micrographic surgery where normal tissue should be conserved. High sensitivity should be sought for in clinical approaches where the goal is to have wide margins to prevent aggressive growth, and where tissue conservation is of lesser importance. Throughout all variations of the test, hair follicles and epidermis were most likely to be misclassified.
The largest limitation of Raman spectrometry as a form of biophysical cancer analysis was the length of time required for data acquisition. For a sample with a size of 1 mm squared, 10 to 20 hours were required. Future modifications to the imaging technique could allow for faster analysis.
Overall, Raman spectrometry has great potential for biophysical cancer analysis. Being able to identify regions of cancerous tissues through this imaging technique will allow for the designation of appropriate margins for resection. By improving surgeries involving the resection of cancerous tissue, the rate of recurrence of the cancer will decrease. This technique could also be used further for more cancers besides basal cell carcinomas.
To read more about this research, the paper can be found here.