Why do We Need Deformable Mirrors?
Deformable mirrors are specialty mirrors that are capable of deforming their reflective surface and thus achieve wavefront correction of optical aberrations. In astronomy it has become an indispensable tool for taking crisp images of distant objects. Astronomy has recently seen huge improvements in the image quality of deep space objects due to clever use of such advanced photonics technologies.
Astronomers typically use ground-based telescopes to search for the deepest and darkest objects. However, these telescopes do come with their own huge disadvantage. Have you ever seen twinkling stars? They might be romantic during a late-night picnic, but they’re absolutely devastating to researchers.
The “twinkling” we see is a result of turbulence in the air, which can make images wavy and blurry. As a result, high-power telescopes are typically built-in dry deserts and high altitudes to reduce the density of molecules that might interfere with the image. Well-funded researchers use space telescopes that are in orbit around the Earth to capture the darkest of objects without interference from the atmosphere. However, due to their expensive price tag and limited reparability, most astronomers typically use ground-based telescopes to find distant galaxies and stars. So they turn to the use of clever electronic optics to counter the effects of the atmosphere.
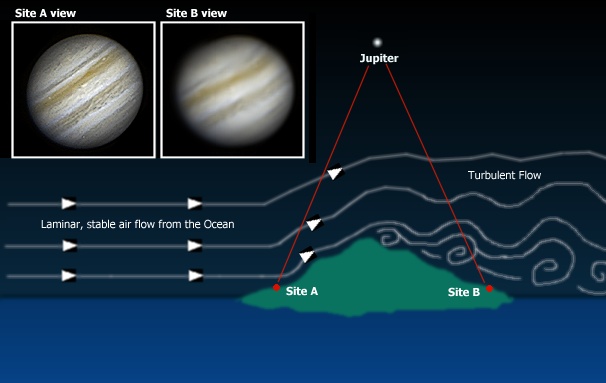
How the difference in turbulence can affect image quality. Courtesy of Views of the Cosmos
Different Imaging Sensors
Your eyes are typically used to see the deep space object. However, our retina can only pick up so much light, so we turn to computers to process images. There are two sensors that are most commonly used to capture images, complementary metal oxide semiconductor (CMOS) and charged coupled devices (CCD). CMOS sensors are typically used in phones and professional cameras. These sensors are composed of individual pixels to sense light intensity and color which are then processed into one large image.
However, in astronomy, to better detect the dimmest light that an object is emitting, scientists use advanced CCD sensors. These sensors read in columns instead of individual pixels. Since they read data at a larger scale without individual pixel boundaries, CCD sensors are less susceptible to noise, which is why they are better for low light situations where every photon of light is critical for composing an image to analyze.
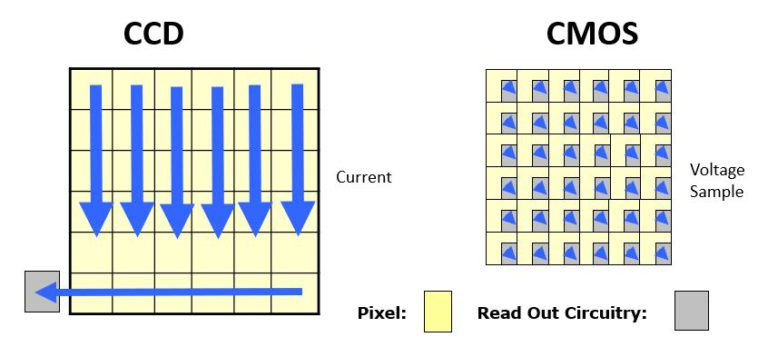
Operations of CCD and CMOS sensors. Courtesy of Automated Imaging Association
Now we know how telescopes capture images, but how do we make these images sharper? Astronomers have developed a clever method of creating “fake” stars with lasers in order to correct for air turbulence and reduce distortions. So how do we create these fake stars with laser?
Fiber Lasers are Used to Generate Guide Stars in Space
In astronomy, a sodium guide star is created by emitting a laser into the sky at a wavelength of 598.2 nm with 10-50 W of power. At this wavelength, the sodium left behind from deteriorated meteorites accumulates in a layer of the mesosphere which is around 50-65 mi in altitude. The energy from the laser will react with the sodium and release fluorescence light. Originally, scientists used dye-based lasers to create these guide stars which took about 50W of power and used organic liquid dye. However, in order to make adjustable optics even better, they turned to another technology that only uses 10W.
The Lawrence Livermore National Laboratory (LLNL) was one of the first researchers to develop a fiber-based sodium laser that was five to ten times more efficient. Similar to dye-based lasers, they used a power source to supply atoms with the required energy to fluoresce. In this laser setup, attached to the fiber is a grating, which reflects certain wavelengths and can be used to filter out one wavelength to allow the material in the doped fiber to absorb it.
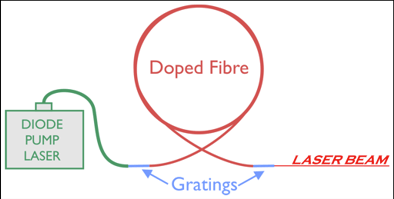
How fiber lasers operate to create. Together with deformable mirrors they enable clear imaging of distant space objects. Courtesy of University of Southampton
Observatories were able to operate more efficiently, and due to the clarity and sharpness of the laser, this consequently resulted in images that were clearer and sharper. They accomplished this by using a nonlinear crystal and combining a 938 nm and 1,583 nm laser to create one uniform 598 nm laser, which is ideal for inducing fluorescence from sodium 50 mi away.
Deformable Mirrors
This light created from the sodium star will slice through the atmosphere and get distorted along its path, bending and refracting. This is then detected by the telescopes and since we know what the guide star originally looks like, we can correct this distortion. The information about the distortion is sent to a supercomputer where commands are sent out to two types of deformable mirrors to reverse the effects. One type of adapting mirror is when one continuous thin piece of reflective material is adjusted in multiple areas simultaneously. Another method is to actuate and adjust individual pieces of the entire mirror, though this is not suitable for astronomical applications, as the segments create boundary grains that can negatively affect image quality.

Individual (left) vs Continuous (right) mirror adjustments. Image courtesy of RP Photonics Encyclopedia
Conclusion
Laser-guided and deformable mirrors have enabled scientists to create the sharpest images possible given current technology and advancements. From creating more efficient and sharper lasers to precise deformable mirrors, astronomers have used these advanced photonics to enhance images to previously unimaginable resolutions. Researchers won’t stop here as future plans are already developing to add multiple precise emitters which will produce even sharper images as you sample more parts of the sky. By using a laser to guide astronomers to exponentially better images, they can unlock the secrets of the universe.
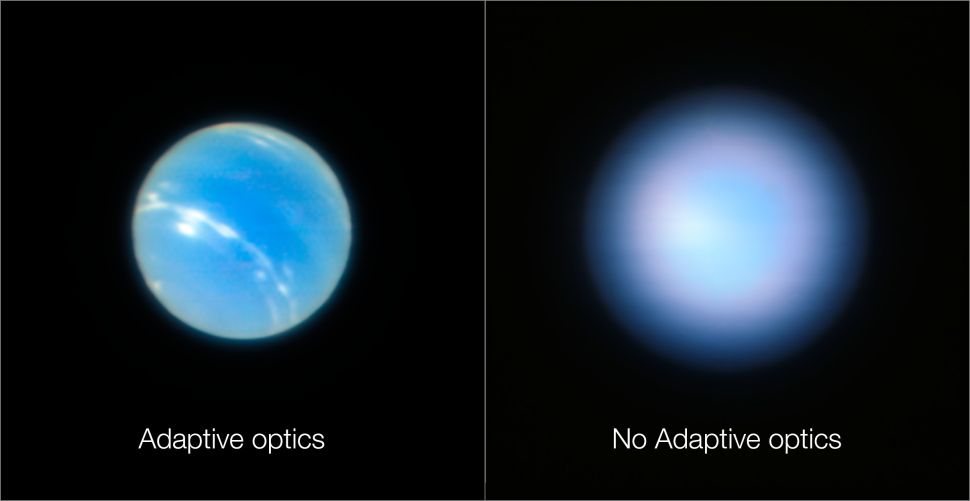
The difference between adaptive optics and regular view. Image courtesy of Space.com
This post was brought to you by DataRay Inc - the masters of Laser Beam Profilers