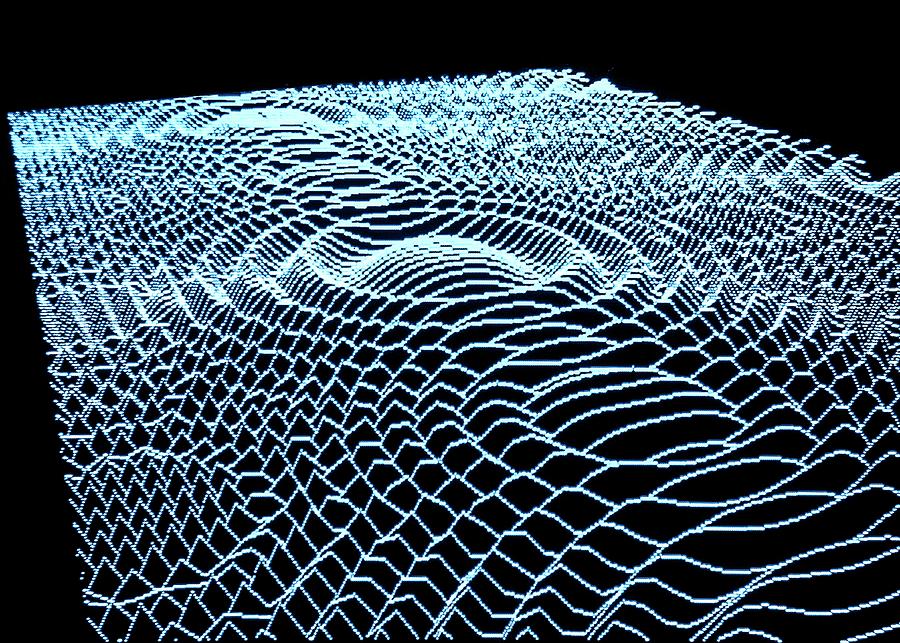
Digital holography provides quantitative images that are label-free and high-contrast. Today, digital holographic interferometry presents a high-resolution technique for imaging small features on living cells. Courtesy of Pixels.
High Resolution Microscopy
Image quality in high-resolution optical microscopy is predominately restricted by the diffraction limit– a fundamental consequence of using lenses. High-resolution microscopy can be categorized by two distinct methods: surface (including surface plasmon resonance) and three-dimensional. The latter presents opportunities to image beyond the diffraction limit, as we can gather information not available with standard optical techniques. Interferometry, for example, is capable of recording a sample’s phase as well as its amplitude. Holography in particular can process extremely small features in real-time; digital holography has successfully characterized properties of living cells such as thickness, viability, and migration. Significant studies include reconstructing pancreatic tumor cells and imaging living neurons.
Interferometry and Digital Holography
Interferometric imaging methods like holography record information via an interference pattern. The interference in a holograph forms when two beams, a reference beam and the object beam, meet at a the face of a CCD camera. The beams emit from the same source but the reference beam travels parallel with a fixed path length while the object beam interacts with the sample. Digital holography then utilizes algorithms to convert the CCD signal into a three-dimensional holographic image of the sample. Digital holographic microscopy (DHM) is diffraction-limited; it will often lose information about the sample as the reconstruction appears unfocused within the hologram. This typically limits DHM to applications with a short working distance and small field-of-view.
A collaborative research team in Aquascalientes and Jalisco, Mexico modified the traditional DHM setup to successfully image biological samples via another nondestructive measurement technique. The transmission digital holographic interferometry (t-DHI) system greatly reduces spherical aberrations, thereby overcoming application limitations. Their paper published in Optics Express shows how they validated the method by comparing results of their interferometric approach with those of a commercial confocal microscope.
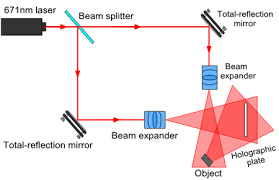
A basic diagram of a holographic imaging setup. Notice that digital holography uses a sCMOS sensor instead of the holographic plate. Courtesy of CNI Laser.
There are two types of digital holography that describe the sample beam’s interaction with the sample. Transmission holography records the optical path difference between reference and sample beam, while reflection holography measures the reflected wavefront from the sample. The research team chose to develop the transmissive method because transmission DHM system has successfully imaged live cells via quantitative phase measurement. This non-invasive technique would allow researchers to study cells long-term without disturbing their function or development.
Digital Holography Method
The research team describes their t-DHI configuration as a “two beam and two object-state optical technique.” The reference and object beam overlap so that they are recorded simultaneously, and the technique requires two image holograms to determine the optical phase difference. Here we have a reference hologram and a second hologram recorded after the sample is displaced. The retrieved phase variation is not directly related to the object displacement, but instead to the object beam’s interaction with the sample of interest.
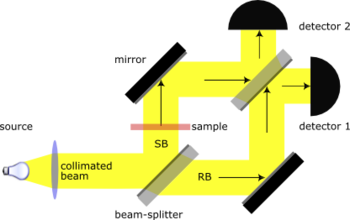
A traditional Mach-Zender setup commonly used in DHM applications. Courtesy of Wikipedia.
Digital Holography Setup
Digital holography systems typically use a Mach-Zender layout. A standard Mach-Zender uses mirrors to reflect the reference and object beams so that they both pass through a half-silvered mirror before entering two detectors. Only one detector will receive light if there is no sample in the path, as the sample and reference beam arrive a half wavelength out of phase with each other. When a sample is placed in the path of the object beam, it can be reconstructed by comparing the intensity measurements on both detectors.
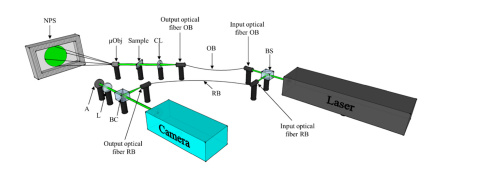
The research team’s t-DHI configuration. Courtesy of Optics Express.
The controlled magnification t-DHI system utilizes a 532nm, 200mW laser source. An 80:20 non-polarizing beamsplitter divides the initial beam to produce the object and reference beams. Both beams use a spatial filter to couple into a single mode fiber. The output object beam is collimated before traveling through the sample and into an infinity-corrected microscope objective. The objective expands the light toward a phase screen to establish controlled geometric magnification. The projection on the screen focuses onto the camera sensor where it finally interferes with the reference beam. A sCMOS sensor records the interference pattern. This configuration avoids diffraction effects while also removing spherical wavefront distortion.
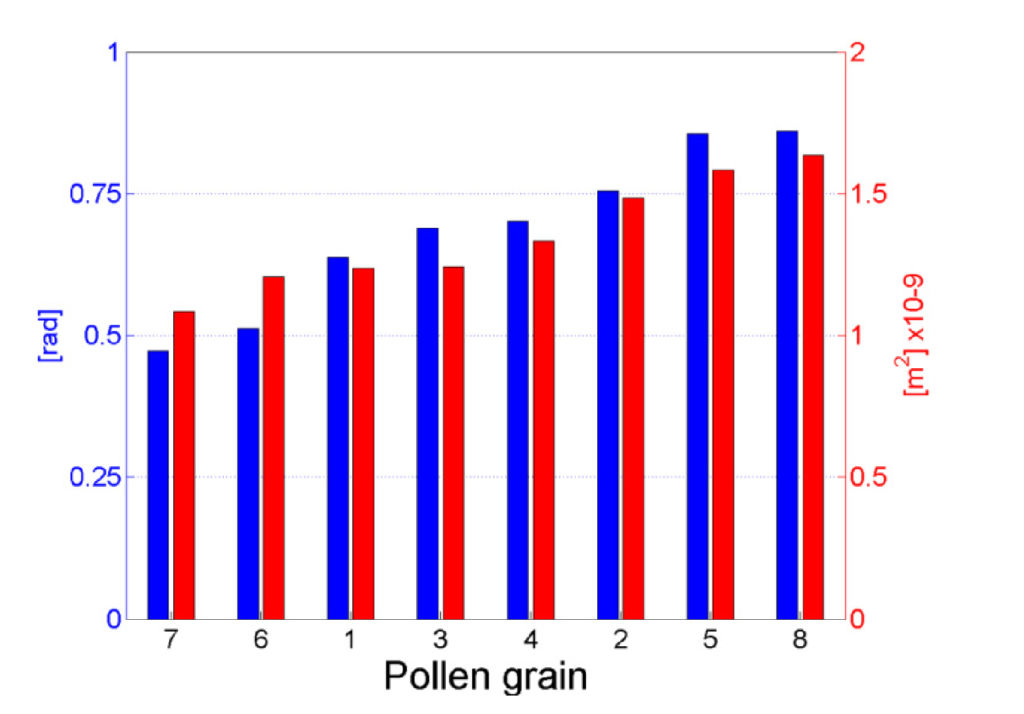
The area and optical phase intensity measurements for each pollen grain. Courtesy of Optics Express.
The Experiment
The team tested their digital holographic interferometry system on pollen grains because the biological sample is well-characterized and semitransparent. They used an optical microscope to evenly distribute and fix the grains to a slide, and a second slide sits above the sample to prevent wavefront distortion off the solidified fixing solution. A customized algorithm facilitates direct comparison between optical phase information of the interferometric signal and the confocal measurements. The researchers applied a digital image processing algorithm to the confocal image to obtain the area of each grain; a second algorithm retrieves optical phase intensities from the unwrapped phase map of the digital holograph.
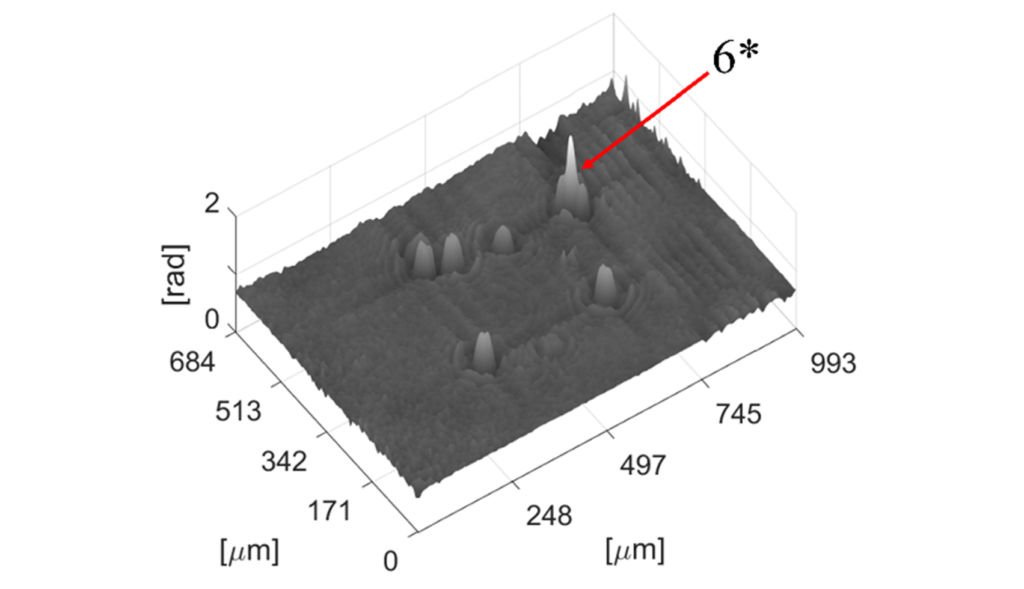
The 3D plot from the unwrapped phase map shows the overlapping intensities at particle 6. Courtesy of Optics Express.
If you are enjoying this article, you might also like our Complete Guide to 3D Cameras.
The Results
Initial results of the first pollen fixation arrangement confirmed the correlation between size and phase measurements. Namely, we see pollen grain “8” has the largest intensity value and the greatest area, while grain “7” is smallest in area and intensity. The researchers then analyzed the grains in three additional arrangements. They found that the t-DHI image shows overlapping the phase map when grains were placed close together. This is recorded as the sum of the intensities from each grain rather than marking them individually. An additional algorithm, however, can estimate the intensity contribution of each particle operating under the assumption of a direct relationship between area and intensity. In the final PFA, the researchers show that the confocal microscope imaged two grains to be very similar in size, but they could be differentiated via the optical phase.
Moving Forward with Digital Holography
This t-DHI system produces a smooth phase magnitude map that directly correlates with the dimensions of a sample of interest. According to the research team, theirs is the first digital holographic interferometry system to successfully measure semitransparent, biological samples. Employing an interferometric setup over the more traditional microscopy configuration allowed the researchers to greatly improve upon imaging abilities for extremely small features by eliminating wavefront distortion and diffraction effects. Their work exhibits that t-DHI could soon open to many more applications that include inorganic sample studies.
For more on their method and setup, find the team’s account of their transmission digital holographic microscopy system here.