Welcome to the intricate and expansive universe of the electromagnetic spectrum, a fundamental yet often overlooked cornerstone of modern science and technology. Spanning from the extreme ultraviolet (XUV) rays, barely perceptible yet instrumental in shaping the very fabric of our cosmos, to the familiar yet complex microwaves and millimeter waves, which silently orchestrate much of our daily digital dance.
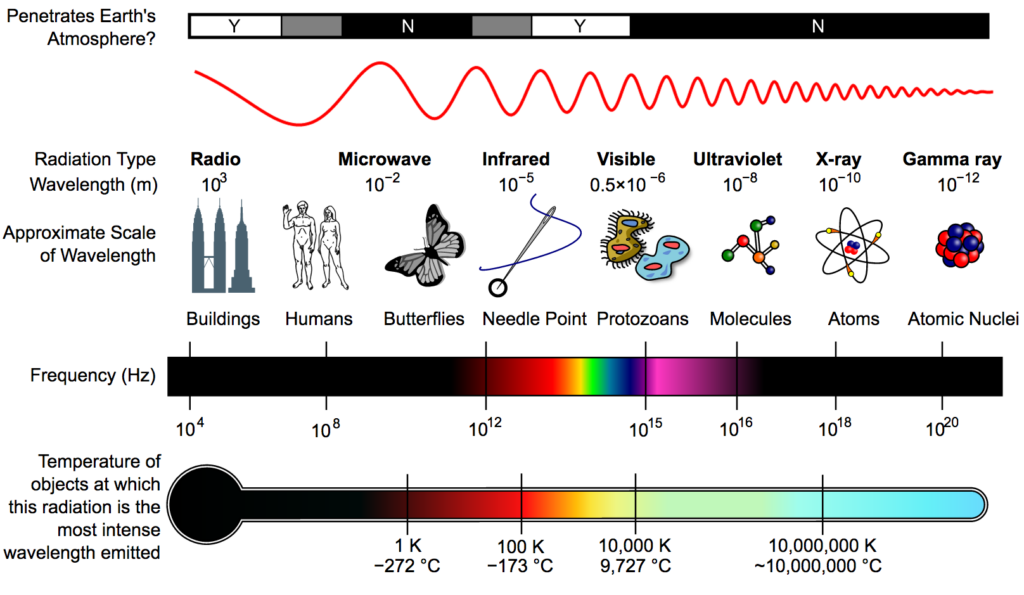
Scaling the Electromagnetic Spectrum: Wavelengths, Frequencies, and Real-World Comparisons. Image courtesy from Wikipedia.
This spectrum, a continuum of electromagnetic waves, is not just a scientific abstraction but a vivid tapestry woven into the very core of our existence. Each wavelength, from the minuscule to the massive, carries a unique story, echoing through space and time, influencing everything from the smallest quantum particles to the grandest galaxies. As we embark on this journey through the electromagnetic spectrum, prepare to uncover the hidden symphony of waves that underpin our universe, shape our technologies, and subtly mold our everyday experiences. This exploration is not just about understanding a range of frequencies; it’s about decoding the language of the universe, a language spoken in the tongues of photons and permeating through the vastness of the cosmic expanse.
This article is brought to you by LECC Technology - Pioneering Laser Technology for over 20 years.
1. Extreme Ultraviolet (XUV) Radiation within the Electromagnetic Spectrum
Definition and Position in the Spectrum: Extreme Ultraviolet (XUV) radiation represents a portion of the electromagnetic spectrum that lies at the boundary between X-rays and ultraviolet light. Characterized by wavelengths ranging from approximately 10 to 121 nanometers, XUV radiation occupies a unique position in the spectrum, bridging the gap between the energy levels of UV radiation and the more energetic X-rays. This range, though less explored, plays a critical role in various scientific and technological fields due to its high energy and penetrating ability.
Natural and Artificial Sources: In the realm of natural sources, the Sun is a significant emitter of XUV radiation, although much of it is absorbed by the Earth’s atmosphere, specifically the ionosphere. This absorption process is crucial for protecting life on Earth from the potentially harmful effects of high-energy radiation. In space, XUV radiation can be observed from various astronomical phenomena, including solar flares and the corona of stars, offering insights into stellar compositions and dynamics.
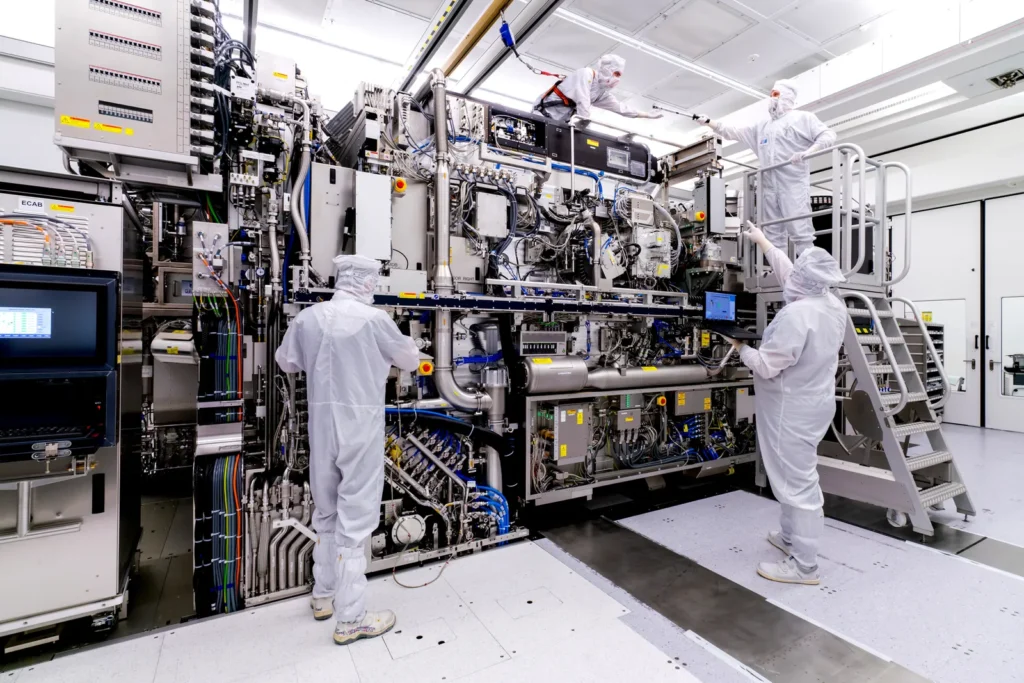
ASML’s EUV Lithography System: The $150M+ Heart of Semiconductor Innovation. Photo Courtesy of ASML.
Artificially, XUV radiation is generated through specialized equipment like synchrotrons and free-electron lasers. These high-energy light sources produce controlled XUV radiation for experimental purposes, allowing scientists to probe the atomic and molecular structures of materials with unprecedented precision.
1.1. Extreme UV Applications in Semiconductor Manufacturing
In the semiconductor industry, XUV radiation is used to create extremely fine patterns on silicon wafers, a critical step in the fabrication of integrated circuits. The shorter wavelength of XUV allows for the creation of smaller, more efficient, and faster electronic components, pushing the boundaries of miniaturization and performance in the semiconductor world.
The evolution of nanometer (nm) technology in semiconductor manufacturing has been a journey marked by remarkable innovation and relentless pursuit of miniaturization. In the late 20th century, the industry standard for circuit feature sizes was in the range of several hundred nanometers – a scale already impressively small by the standards of the time. However, as the principles of Moore’s Law predicted, the drive for greater efficiency, speed, and capacity in electronic devices spurred the industry to push these boundaries even further.
In the early 2000s, the transition to feature sizes below 100 nm marked a significant milestone, ushering in a new era of microprocessor performance and efficiency. As we progressed through the decade, the industry achieved feats that once seemed impossible, moving to 45 nm, 32 nm, and beyond. Each leap in technology brought about more powerful and energy-efficient processors, paving the way for the advanced smartphones, computers, and other electronic devices we rely on today.
Today, we stand at the forefront of another revolutionary shift with the advent of 7 nm, 5 nm, and even smaller feature sizes, largely made possible by the advent of sophisticated technologies like ASML’s EUV lithography systems. This incredible shrinking scale allows for billions more transistors to be packed onto a single chip, exponentially increasing computational power and efficiency. The implications of this advancement are profound, not only continuing the trend of more powerful consumer electronics but also enabling leaps in fields like artificial intelligence, quantum computing, and advanced data processing.
2. Ultraviolet (UV) Radiation: The Invisible Light
Overview and Categorization: Ultraviolet (UV) radiation, a vital segment of the electromagnetic spectrum, occupies the range between visible light and X-rays, with wavelengths from about 10 nm to 400 nm. It is commonly categorized into three types based on its wavelength: UVA (315-400 nm), UVB (280-315 nm), and UVC (100-280 nm). Each type has different properties and interacts with matter in distinct ways. UVA, known for its longer wavelengths, constitutes the majority of UV radiation reaching the Earth’s surface. UVB, with shorter wavelengths, is partially absorbed by the ozone layer but is responsible for skin tanning and sunburn. UVC, the shortest and most energetic UV rays, are almost entirely absorbed by the Earth’s atmosphere and ozone layer.
Benefits and Risks: UV radiation presents a unique balance of benefits and risks to human health and the environment. On the beneficial side, UVB is essential for the synthesis of vitamin D in the skin, crucial for bone health and immune function. UVA, while less intense, contributes to skin aging and can penetrate deeper into the skin layers.
However, excessive exposure to UV radiation, particularly UVB, poses significant health risks, including skin cancer, cataracts, and immune system suppression. It’s this dual nature of UV radiation that necessitates careful management in both personal exposure and in various applications.
2.1. Applications of UV Radiation in Various Contexts
In medical contexts, UV radiation is used for its germicidal properties, especially UVC, in sterilizing equipment and environments. UV light therapy is also employed in treating skin conditions such as psoriasis and vitiligo.
In industrial applications, UV radiation is utilized in curing inks, coatings, and adhesives due to its ability to initiate chemical reactions rapidly. This process is fundamental in printing, automotive, and electronics industries.
The forensic field also leverages UV light. Crime scene investigators use it to reveal blood, fingerprints, and other evidence not visible under regular light. In this way, UV radiation becomes a critical tool in uncovering hidden details that are key to solving crimes.
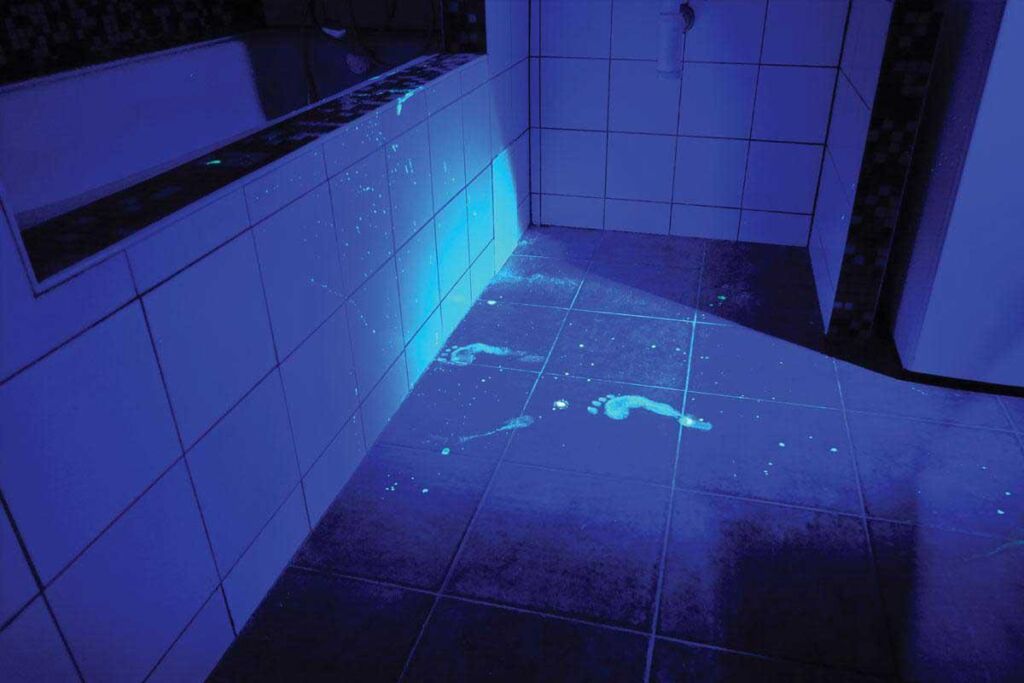
Revealing the Hidden: Forensic UV Light Unveils a Key Clue. Photo courtesy of NightSearcher
The UV range of the electromagnetic spectrum, therefore, is not only a subject of scientific fascination but also a domain of immense practical significance, balancing its inherent risks with a wide array of beneficial applications across various fields.
3. Visible Light in the Electromagnetic Spectrum
Spectrum and Human Perception: Visible light, the most familiar part of the electromagnetic spectrum, is the range of wavelengths perceptible to the human eye, typically spanning from about 380 to 750 nanometers. This spectrum manifests as the colors we see every day: violet, blue, green, yellow, orange, and red. Each color corresponds to a different wavelength, with violet having the shortest wavelength and red the longest. The human eye’s sensitivity to these wavelengths is not just a biological phenomenon but also a cornerstone of our visual perception, shaping our interaction with the world around us.
Sources and Everyday Applications: The primary natural source of visible light is the Sun, which illuminates our planet and is essential for life. Artificial sources include incandescent bulbs, LEDs, and lasers, each playing a crucial role in modern life. Visible light is integral to a myriad of applications: it aids in the growth of plants through photosynthesis, provides illumination for our activities, and plays a significant role in art and design, influencing aesthetics and mood.
Visible light’s interaction with materials—absorption, reflection, refraction—is fundamental in fields ranging from photography to the operation of optical instruments like microscopes and telescopes.
3.1. Applications of Visible Light in Communication Technologies
In communication technologies, visible light has a particularly transformative role. Fiber optics, a technology that uses light to transmit data, has revolutionized telecommunications. Optical fibers, made from glass or plastic, guide light along their length, allowing for high-speed data transmission over long distances with minimal loss. This technology is the backbone of internet and telephone networks, enabling rapid, global communication.
The use of visible light in communication extends to more cutting-edge applications as well, such as Li-Fi (Light Fidelity), which uses visible light for wireless data transmission, offering an alternative to traditional Wi-Fi with potentially higher speeds and improved security.
In conclusion, visible light, though just a small part of the electromagnetic spectrum, is immensely powerful. It not only enables us to see the world but also connects us to it in ways that are both fundamental and profoundly innovative.
4. Infrared (IR) Radiation and the Electromagnetic Spectrum
Types and Placement in the Spectrum: Infrared (IR) radiation lies just beyond the visible spectrum, with wavelengths longer than red light and shorter than microwaves, typically ranging from 700 nanometers to 1 millimeter. It is commonly divided into three categories: near-infrared (NIR), from about 700 nm to 1.4 μm; mid-infrared (MIR), from about 1.4 μm to 3 μm; and far-infrared (FIR), extending from 3 μm to 1 mm. Each type of IR radiation interacts with matter in different ways, leading to a variety of applications. NIR, closest to visible light, is often used in electronic devices, while MIR and FIR, with their longer wavelengths, are more associated with thermal properties.
Sources and Applications: The primary natural source of IR radiation is heat or thermal radiation. Any object that has a temperature emits infrared radiation, with the Sun being a significant natural source. Artificial sources include heaters, remote controls, and specialized LEDs and lasers used in various technological devices.
Infrared technology has wide-ranging applications. In thermal imaging, IR radiation is used to detect heat patterns and is invaluable in fields like firefighting, building inspection, and medical diagnostics. Infrared cameras can reveal heat leaks in buildings, identify overheating electrical components, and provide detailed images of the human body’s thermal patterns, aiding in disease diagnosis.
In the field of astrophysics, IR telescopes allow astronomers to observe distant celestial objects obscured by cosmic dust. Since infrared light can penetrate dust clouds that block visible light, it opens a window into regions of space that are otherwise hidden, such as the center of galaxies and star-forming regions.
4.1. Everyday Encounters of IR Radiation
In everyday life, we encounter IR radiation more often than we might realize. The warmth we feel from sunlight, the heat from a stove, and even the human body are all sources of infrared radiation. Remote controls for TVs and other electronic devices use IR LEDs to transmit signals. In newer technologies, IR is used for facial recognition in smartphones and for secure, short-range communication in devices like wireless headphones and computer peripherals.
In conclusion, infrared radiation, an integral part of the electromagnetic spectrum, not only offers a window into the unseen thermal world but also has numerous practical applications that impact our daily lives and scientific understanding.
5. Microwaves: The Workhorses of Communication
Definition and Characteristics: Microwaves, a significant part of the electromagnetic spectrum, are characterized by wavelengths ranging from about 1 millimeter to 1 meter, situated between infrared radiation and radio waves. They are distinguished by their longer wavelengths compared to infrared and visible light but shorter than those of radio waves. This unique positioning in the spectrum endows microwaves with distinct properties, such as their ability to penetrate various materials, making them highly valuable in multiple fields. Microwaves are also known for their resonance with water molecules, which is fundamental to their most well-known application in heating food.
Role in Communication, Industry, and Domestic Appliances: Microwaves electromagnetic waves play a pivotal role in communications. They are the backbone of modern wireless communication, used in everything from satellite transmissions to Wi-Fi networks. Due to their ability to travel long distances and penetrate atmospheric obstacles, they are ideal for point-to-point communication systems and are crucial in broadcasting, cellular networks, and satellite communication.
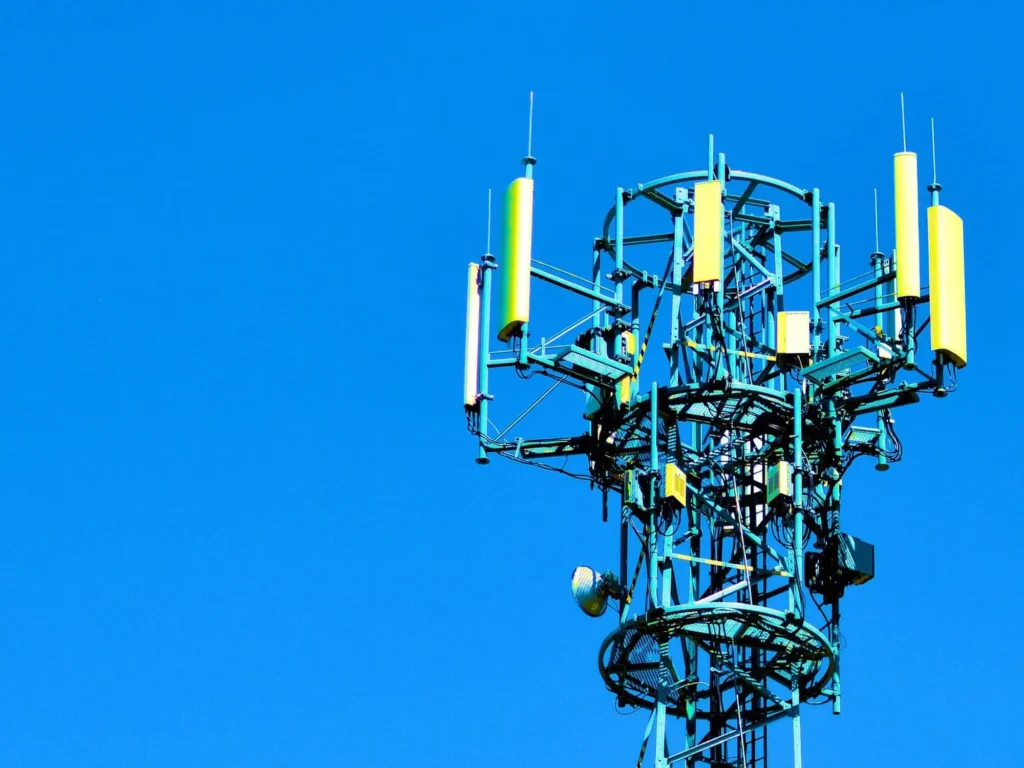
The Backbone of Wireless Communication: A Cell Tower in Operation.
In the industrial sector, microwaves have unique applications in processing materials. They are used for drying and curing products, particularly in the chemical and food industries. Their ability to heat substances quickly and uniformly makes them efficient for these processes.
The most familiar domestic application of microwaves is in microwave ovens. These appliances use microwave radiation to agitate water molecules in food, generating heat through this friction and cooking the food rapidly and efficiently.
5.1. Emerging Technologies Utilizing Microwaves
Emerging technologies continue to find novel uses for microwaves. In the medical field, microwave ablation is becoming a more common, minimally invasive cancer treatment, using targeted microwaves to heat and destroy cancer cells.
In the area of energy production, research is ongoing in using microwaves for more efficient solar power conversion and potentially for wireless power transmission, a concept that could revolutionize the way we access electrical power.
Moreover, the field of radar technology, which has traditionally used microwaves for navigation and weather forecasting, is continually advancing. Modern developments include high-resolution imaging radars for autonomous vehicles and enhanced air traffic control systems.
In conclusion, microwaves, with their unique position in the electromagnetic spectrum, are not just confined to kitchen appliances; they are a fundamental component in communication, industrial processes, and a host of emerging technologies, showcasing their versatility and importance in both our everyday lives and cutting-edge scientific applications.
6. Millimeter Waves and their Place in the Electromagnetic Spectrum
Definition and Spectrum Position: Millimeter wave (mmWave), also known as the millimeter band, occupies a specific portion of the electromagnetic spectrum with wavelengths that range from 10 millimeters (30 GHz) to 1 millimeter (300 GHz). This range is also referred to as the extremely high frequency (EHF) band by the International Telecommunication Union (ITU). Positioned between the more common microwave frequencies and the terahertz radiation band, millimeter waves offer a unique blend of properties due to their wavelength and frequency characteristics.
Advantages and Applications: Millimeter waves are utilized in a broad spectrum of products and services, notably in high-speed, point-to-point wireless local area networks (WLANs) and broadband access. In telecommunications, they are crucial for enabling higher data rates compared to lower frequencies used for traditional Wi-Fi and cellular networks. The short propagation distance of millimeter waves is instrumental in the design of small cell networks, facilitating higher bandwidth allocation per user and efficient channel reuse across the coverage area.
The compact size of antennas suitable for millimeter wave frequencies makes them ideal for integration into small Internet of Things (IoT) devices. This is particularly relevant in developing applications that require minimal form factors without compromising communication capabilities.
Challenges and Limitations: One of the key limitations of millimeter waves is their susceptibility to absorption by gases and moisture in the atmosphere, leading to reduced range and signal strength, a phenomenon known as rain fade. Their propagation distance is shorter compared to lower frequency bands, ranging from a few meters to a kilometer. Furthermore, millimeter waves travel predominantly by line of sight and are easily blocked or degraded by physical obstacles like buildings, foliage, and even the human body.
6.1. Emerging and Current Uses of mm Waves
Despite these challenges, millimeter waves are employed in various innovative applications. They are essential in the functioning of short-range radar systems and security scanners, like those used in airports. In the telecommunications sector, they play a pivotal role in high-bandwidth WLANs and short-range personal area networks (PANs). The high bandwidth capacity of millimeter waves is ideal for applications such as wireless transmission of ultra-high definition video and communications from low-power IoT devices. Additionally, their properties are highly suitable for facilitating communication between autonomous vehicles.
Millimeter waves are being harnessed in several key technologies and standards, including IEEE 802.15 for wireless PANs, IEEE 802.16 (WiMAX) for wireless metropolitan area networks, IEEE 802.11ad for multiple gigabit wireless systems at 60 GHz, and 5G cellular telecommunications in the 24 GHz to 39 GHz bands.
In comparison with other spectrums, Wi-Fi currently utilizes frequencies in the 2.4 GHz, 5 GHz, and 6 GHz bands, which are known as microwave bands. Cellular networks use frequencies in the 600 MHz to 700 MHz and 2.5 GHz to 3.7 GHz bands. These bands propagate farther than millimeter wave but support lower bandwidths, highlighting the unique position and potential of millimeter waves in the electromagnetic spectrum.
7. Summary
As we conclude our exploration of the electromagnetic spectrum, let’s reflect on the key insights from each segment and their collective impact on technology and daily life.
Extreme Ultraviolet (XUV) Radiation: We began with the XUV range, highlighting its crucial role in scientific research and technology, particularly in astronomy and semiconductor manufacturing. Its ability to reveal the unseen and aid in creating intricate electronic components underscores its scientific significance.
Ultraviolet (UV) Radiation: Next, we examined UV radiation, balancing its benefits, like vitamin D synthesis, against its risks, including skin cancer. Its applications in sterilization and forensic science demonstrate its practical utility beyond its natural occurrence.
Visible Light: The spectrum of visible light, fundamental to human perception, plays a vital role in everything from the beauty of art to the functionality of optical technologies. Its ubiquitous presence in our lives is a testament to its importance.
Infrared (IR) Radiation: Infrared radiation, with its diverse applications in thermal imaging, remote controls, and astrophysics, highlights how different wavelengths serve varied and specific purposes, from everyday convenience to exploring the cosmos.
Microwaves: The role of microwaves in communication and cooking illustrates the versatility of the electromagnetic spectrum. From powering our smartphones to heating our meals, microwaves are an integral part of modern life.
Millimeter Waves: Finally, millimeter waves, crucial in 5G technology and security screening, represent the forefront of technological advancement, offering new possibilities in high-speed communication and safety.
The Interconnectedness of the Spectrum: Each part of the electromagnetic spectrum, from XUV to millimeter waves, plays a unique role, yet they are all interconnected in their contribution to technological advancement and everyday life. Understanding this spectrum is not just an academic pursuit but a window into the forces that shape our world and drive innovation.
7.1. Encouraging Exploration and Engagement
This journey through the electromagnetic spectrum is just the beginning. We encourage you to delve deeper into these fascinating wavelengths, be it through academic study, visiting science museums, or simply by staying curious about the technological marvels that surround us. Whether it’s observing the stars, understanding how your smartphone connects to the world, or appreciating the invisible waves that heat your food, there’s a vast universe of discovery within the electromagnetic spectrum waiting to be explored.
Engage more with this subject, and you’ll find yourself at the crossroads of science, technology, and daily life, where the invisible becomes visible, and the unimaginable becomes possible.
8. References and Further Reading
Academic and Scientific Resources: below are some texts that cover some fundamental ground on electromagnetic waves:
- “Fundamentals of Photonics” by Bahaa E. A. Saleh and Malvin Carl Teich: A comprehensive textbook offering an in-depth understanding of photonics, including detailed sections on various parts of the electromagnetic spectrum.
- “Introduction to Electrodynamics” by David J. Griffiths: This classic text provides a thorough exploration of electrodynamics, with a focus on the fundamentals of electromagnetic theory.
- “The Feynman Lectures on Physics, Vol. II: The Electromagnetic Field” by Richard P. Feynman: A seminal series offering an insightful perspective on electromagnetic fields, ideal for those looking to deepen their understanding of physics.
Books and Articles for Extended Learning: We list some books below to read for further enrichment on the nature of EM waves and history:
- “The Invisible Rainbow: A History of Electricity and Life” by Arthur Firstenberg: This book explores the relationship between electricity, electromagnetic fields, and life, providing a historical perspective.
- “Electromagnetic Fields and Waves” by Vladimir Rojansky: An accessible book that delves into electromagnetic fields and waves, suitable for those with a foundational knowledge of physics.
- “Light: A Very Short Introduction” by Ian A. Walmsley: Part of the Very Short Introductions series, this book offers a concise and readable overview of the nature of light and its role in the electromagnetic spectrum.
- “Microwave Engineering” by David M. Pozar: A key text for understanding microwaves and their applications, particularly in telecommunications and radar systems.
These resources offer a mix of foundational knowledge and up-to-date scientific information. They should provide the readers with a comprehensive understanding of the electromagnetic spectrum and its vast significance in both academic and practical contexts.
If you liked this article, you might also like our recent post on Neutrino Oscillations.
I was a little surprised that the gammaray spectrum wasn’t discussed since the 1.0216 Mev "threshold" gammaray is the energy at which free electron-positron pairs can be produced. This mechanism is also responsible for neutron beta-decay and can produce a stable proton, which in turn can attract a free electron, from the beta-decay, to create (the first) simple hydrogen atom. The shared positron from the decay provides the new proton’s positive charge.
Thank you for highlighting the significance of the 1.0216 MeV gamma-ray energy level and its role in electron-positron pair production. Your point about its connection to fundamental processes like neutron beta-decay and the formation of hydrogen atoms is indeed fascinating and underscores the intricate interplay of particle physics.
Our article aimed to provide a broad overview of the electromagnetic spectrum, which meant that some specialized topics, including the detailed mechanisms within the gamma-ray spectrum, were not covered in depth. Your comment is a valuable reminder of the depth of physics that underlies each segment of the electromagnetic spectrum. We appreciate your insight and will consider exploring these more complex phenomena in future content.