Welcome to our exploration of the most promising emerging battery technologies poised to transform energy storage in the coming decade. This article delves into five innovative battery types that are not just theoretical but are nearing or have begun their journey towards commercial reality. Each section outlined below will introduce you to a different technology, highlighting its potential impacts, benefits, and the challenges it faces. Read on to discover the future of batteries—a future that promises safer, more efficient, and sustainable power solutions for our world.
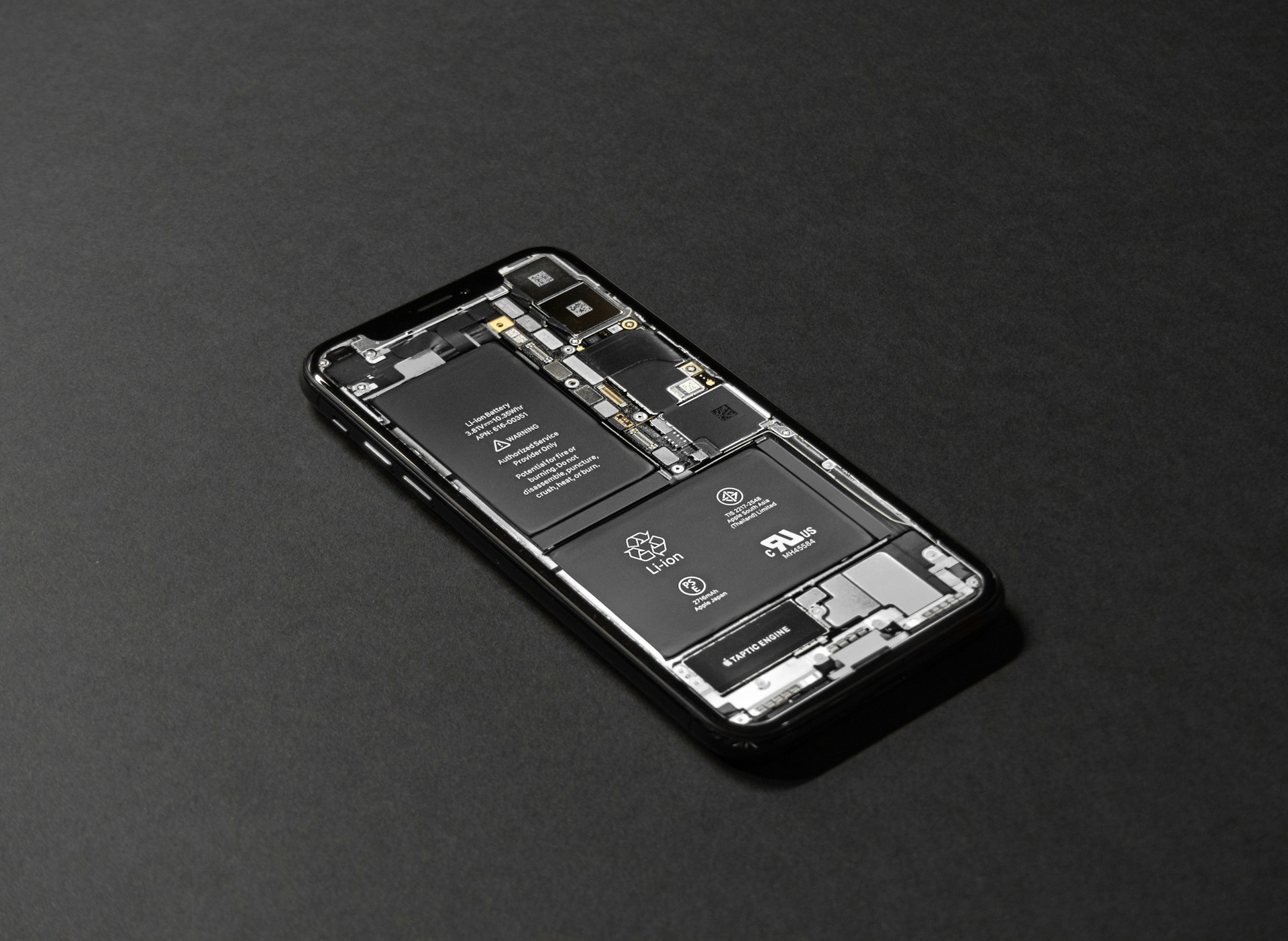
Peek inside a smartphone: The lithium-ion battery that powers our daily communications. Image courtesy of Tyler Lastovich.
Introduction
Batteries are fundamental to modern energy systems, serving as the backbone for everything from mobile devices to electric vehicles and renewable energy storage. As these applications expand, the limitations of current battery technologies become more apparent, driving a critical need for advancements. Industries such as automotive and energy sectors require batteries that are not only more efficient and safer but also environmentally sustainable and economically feasible. This urgent need propels the development of innovative battery technologies that promise to meet the future demands of a rapidly electrifying world. With global energy needs evolving, next-generation batteries are poised to play a pivotal role in enabling a sustainable and efficient future.
The Importance of Emerging Battery Technologies
Current mainstream battery technologies, particularly lithium-ion batteries, are grappling with significant limitations that affect their wider adoption. These include a limited lifecycle of approximately 1,000 to 2,000 charge cycles before significant degradation occurs, lengthy charging times of several hours for full charge in larger applications, and potential safety risks such as thermal runaway, which can lead to fires or explosions. For example, research indicates that the failure rate of lithium-ion batteries is about 1 in 10 million cells, yet this statistic still presents significant risks in high-volume scenarios such as electric vehicles or large energy storage installations.
From an environmental perspective, the lifecycle of lithium-ion batteries poses challenges in mining and disposal. The mining of lithium, for instance, can result in water pollution and depletion, with approximately 500,000 gallons of water used to mine just one ton of lithium. Moreover, less than 5% of lithium-ion batteries are recycled, leading to substantial waste and environmental concerns.
Economically, the demand for raw materials like lithium and cobalt has escalated, causing price fluctuations and supply chain issues. Between 2016 and 2018, the price of lithium carbonate tripled due to surging demand from the battery sector, impacting the cost-effectiveness of lithium-ion batteries.
Performance-wise, while lithium-ion batteries offer an energy density of about 150 to 250 Wh/kg, this is often insufficient for more demanding applications like commercial electric vehicles or grid-scale energy storage, which require longer duration and higher energy capacities.
These challenges emphasize the need for innovative battery technologies that can provide higher energy densities, faster charging times, improved safety, reduced environmental impact, and economic viability. As the world shifts toward more sustainable energy solutions, the role of advanced battery technologies becomes crucial in meeting these evolving demands.
Solid-State Batteries
Solid-state batteries represent a significant advancement in battery technology, utilizing solid electrolytes rather than the liquid or gel electrolytes found in conventional lithium-ion batteries. This change in material composition is poised to address many of the limitations of today’s batteries.
Current Development Status: Solid-state batteries are in various stages of development, with several major automotive and technology companies investing heavily in this technology. As of recent reports, commercial applications are expected to begin appearing in the next few years, with some prototypes already in testing phases for use in electric vehicles and high-end electronics.
Benefits: One of the most notable benefits of solid-state batteries is improved safety. The solid electrolytes are non-flammable, significantly reducing the risk of fires and explosions associated with lithium-ion batteries. Additionally, these batteries can potentially achieve higher energy densities — estimates suggest up to 2.5 times the energy density of current lithium-ion batteries. This could drastically increase the range of electric vehicles and the longevity of consumer electronics without increasing the battery size.
Challenges to Commercial Adoption: Despite their advantages, solid-state batteries face several challenges. The foremost is the cost of production, as current manufacturing processes for solid-state batteries are more complex and expensive than those for lithium-ion batteries. Another challenge is the brittleness of solid electrolytes, which can lead to cracking and reduced life spans under typical usage conditions that involve regular charging and discharging cycles. Moreover, ensuring consistent quality and performance at scale remains a significant hurdle.
Overall, while solid-state batteries offer a promising future with considerable advantages over traditional battery technologies, overcoming these commercialization challenges will be critical to their widespread adoption and success.
Lithium-Sulfur Batteries
Lithium-sulfur (Li-S) batteries operate on a distinct chemical reaction mechanism compared to conventional lithium-ion batteries. They use a lithium anode and a sulfur cathode, which during discharge, form lithium sulfide through a series of electrochemical reactions. This technology is currently in the developmental stage but shows significant promise due to its theoretical energy density and cost advantages.
Potential Advantages: Li-S batteries can achieve an energy density of up to 500 Wh/kg, which is significantly higher than the typical 150-250 Wh/kg offered by lithium-ion batteries. This higher energy density could dramatically extend the range of electric vehicles and increase the lifespan of portable electronics without increasing the battery’s weight. Additionally, sulfur is abundant and less expensive than other battery materials, which could reduce the material costs by about 30-40% compared to lithium-ion batteries, making Li-S batteries a potentially more economical option.
Technical Hurdles: Despite these advantages, several technical challenges hinder the commercial viability of Li-S batteries. The primary issue is the “shuttle effect,” where sulfur molecules dissolve into the electrolyte and migrate between the electrodes, which gradually degrades the battery’s capacity. Another significant challenge is the volume expansion of the sulfur cathode during discharge, which can cause mechanical stress and break the electrical contacts within the battery. Overcoming these hurdles requires advancements in electrode and electrolyte materials to stabilize the chemical reactions and improve the battery’s overall durability and cycle life.
In summary, while lithium-sulfur batteries offer impressive potential benefits in terms of efficiency and cost, addressing the inherent technical challenges is essential for their advancement from laboratory research to practical, commercial applications.
Sodium-Ion Batteries
Sodium-ion batteries are emerging as a promising alternative to lithium-ion batteries, primarily due to the relative abundance and accessibility of sodium compared to lithium. This shift is particularly relevant as the demand for lithium surges, straining supplies and elevating prices, especially with the proliferation of electric vehicles and large-scale battery storage systems.
Relevance in the Context of Lithium Scarcity: Sodium, unlike lithium, is abundant and widely available, sourced easily from sea water and other saline sources, making it a more sustainable and cheaper alternative in the long run. The widespread availability of sodium could alleviate the supply constraints and geopolitical tensions associated with lithium mining.
Comparison with Lithium-Ion Batteries: Performance-wise, sodium-ion batteries typically offer lower energy densities than lithium-ion batteries—currently achieving about 100-150 Wh/kg compared to the 150-250 Wh/kg offered by lithium-ion counterparts. However, they compensate for this with advantages in safety and stability, as sodium does not pose the same risks for thermal runaway as lithium. Economically, the lower cost of sodium and less complex manufacturing processes could make sodium-ion batteries a more cost-effective option for large-scale energy storage and low-cost consumer electronics.
Current Applications and Future Potential: Sodium-ion batteries are currently being used in low-energy-demand applications such as grid storage, where energy density is less critical than cost and cycle stability. The future potential of sodium-ion technology is significant, particularly in stabilizing renewable energy deployment by providing affordable, large-scale storage solutions. Further research and development could enhance the performance parameters of sodium-ion batteries, expanding their use to more energy-intensive applications like electric vehicles and portable electronics.
In summary, sodium-ion batteries present a viable, economically attractive alternative to lithium-ion systems, particularly in the context of global lithium scarcity and the need for sustainable, safe, and stable energy storage solutions.
Aluminum-Ion Batteries
Aluminum-ion batteries are an innovative class of energy storage devices, leveraging aluminum’s natural abundance and trivalent properties, which facilitate higher charge carriers per ion. This technology differentiates itself with several unique properties, making it an intriguing option in the realm of advanced battery solutions.
Unique Properties and Advantages: Aluminum-ion batteries excel in areas critical for modern technology demands. One of their standout features is the capability for ultra-fast charging. These batteries can potentially be charged to full capacity in less than 10 minutes, significantly faster than current lithium-ion batteries. Moreover, they boast an impressive cycle life, capable of thousands of charge-discharge cycles with minimal degradation, surpassing the typical lithium-ion batteries which offer around 1,000 to 2,000 cycles. This extended lifecycle significantly enhances their economic and environmental appeal by reducing the frequency of battery replacements.
Challenges Limiting Adoption: Despite their potential, several challenges hinder the widespread adoption of aluminum-ion batteries. Currently, the primary issue is the relatively low energy density, which at about 60-100 Wh/kg, falls short of the energy density offered by lithium-ion batteries. This lower energy density limits their immediate applicability in high-energy-demand applications such as electric vehicles. Additionally, existing technologies still face hurdles in electrode material compatibility and stability, which can affect overall performance and efficiency.
The ongoing research focuses on enhancing the cathode materials and the electrolyte formulations to improve energy density and ensure the stability of the battery throughout its lifecycle. Addressing these technological challenges is crucial for transitioning aluminum-ion batteries from a promising concept into a viable commercial product.
Metal-Air Batteries
Metal-air batteries, particularly zinc-air types, are noted for their unique chemistry and potential in high-energy storage applications. These batteries generate electricity through the oxidation of zinc with oxygen from the air, offering higher energy densities than traditional technologies.
Specific Type: Zinc-Air Batteries: Zinc-air batteries utilize zinc as the anode and atmospheric oxygen as the cathode, producing zinc oxide during discharge. This mechanism supports a high energy capacity, leveraging air—a vast and readily available resource. Zinc’s abundance and non-toxic nature make these batteries an appealing choice for sustainable energy storage.
Benefits for High-Energy Storage Applications: With energy densities reaching 300-400 Wh/kg, zinc-air batteries are ideal for applications requiring large energy capacities in compact forms, such as backup power and renewable energy storage. Their potential for mechanical recharging adds versatility across various implementations.
Challenges and Ongoing Research Efforts: The main challenge for zinc-air batteries is their limited rechargeability, attributed to zinc anode instability and dendrite formation during cycling, which diminishes capacity. Also, their performance is highly sensitive to humidity, necessitating advanced sealing and moisture control. Current research focuses on developing more robust air cathode materials and improving electrolyte compositions to enhance battery rechargeability and durability. Innovations in managing air flow and moisture inside the batteries are crucial for advancing zinc-air battery technology toward practical and commercial uses.
Impact of Emerging Battery Technologies on Industries
Emerging battery technologies are set to significantly impact various industries and reshape global energy strategies. Their influence is particularly notable in the automotive and consumer electronics sectors, enhancing efficiency and sustainability.
Impact on the Automotive Industry: Advancements in battery technologies, such as solid-state and lithium-sulfur batteries, will revolutionize the electric vehicle (EV) landscape. For example, solid-state batteries, with their higher energy densities, could potentially double the driving range of EVs compared to current lithium-ion models. This improvement could accelerate the adoption of EVs, aligning with global goals to reduce carbon emissions by decreasing reliance on fossil fuels.
Influence on Consumer Electronics: In consumer electronics, innovative batteries such as aluminum-ion offer faster charging and extended lifecycles, significantly reducing electronic waste and enhancing device sustainability. These attributes could lead to less frequent battery replacements, promoting environmental conservation.
Broader Implications for Global Energy Markets: On a larger scale, technologies like sodium-ion and metal-air batteries could transform energy storage solutions for renewable energy systems. Sodium-ion batteries, in particular, are cost-effective and scalable, ideal for storing excess energy from renewable sources, thereby stabilizing grid performance and supporting sustainable energy transitions.
Sustainability Efforts: These emerging battery technologies also promise substantial environmental benefits. By moving away from scarce and environmentally harmful materials like cobalt and lithium, and improving recyclability, these innovations support sustainability initiatives, helping to reduce global carbon footprints and encourage resource conservation.
As these technologies develop, their adoption will crucially influence economic strategies, environmental policies, and technological advancements worldwide, playing a pivotal role in meeting future energy needs sustainably and efficiently.