The history of glass making can be traced back to at least 3600 BC, perhaps accidentally created from slags of metal-working processes. Since then this transparent material has provided us with beautiful ornaments and sculptures throughout history to delight us. It is interesting how we now use glass that was once used in decorations, to bend and amplify light using fiber amplifiers and transmit webpages on computer screens across continents.
Glass has been used as a medium to bend light, which is shown by inventions such as the prism by Newton, and the bifocal lens by Benjamin Franklin. A few centuries later, guiding light through glass tubes (optical fibers) by total internal reflection was first demonstrated by Daniel Colladen and Jacques Babinet in Paris in the early 1840’s. The first demonstration of light amplification in fibers was shown in 1964 by Koester and Snitzer. Since then the industry has seen a proliferation of the different uses that fiber amplifiers bring in material processing and medicine, with a trend towards higher power outputs and wider spectral regions.
This article will discuss the manufacturing of fibers for amplification, the physics of amplification in optical fibers, and some interesting applications of fiber amplifiers in optical signal processing.
Manufacturing Doped Fiber Amplifiers
Amplification in glass fibers is brought about by the special properties of carefully selected dopants. These dopants are introduced into the fibers during the manufacturing process. Manufacturing these doped optical fibers is done by a method called Modified Chemical Vapor Deposition (MCVD). In MCVD, a rotating drum is heated by a moving flame. The ingredients are passed through the column with a preform being deposited on the sides of the tube. Later, thin fibers are pulled out from the preform to generate fibers of enormous lengths that are rolled onto a drum. The MCVD process is illustrated in the image below.
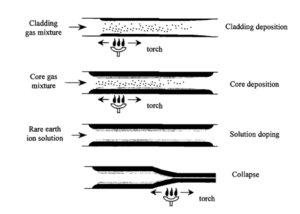
Manufacturing doped optical fibers: Modified chemical vapor deposition (MCVD) for depositing perform for glass fibers. Courtesy ResearchGate.
Initially silicon chloride and certain other gaseous ingredients necessary for the glass composition are passed through the drum. The ensuing reactions at high temperatures (around 1600 C) cause liquid glass to form on the sides. Rare earth ion solution is passed through the tube as dopants. Then on cooling, the glassy state sets in. The rate of cooling, the choice of dopant concentration and the gas ingredients are crucial in determining the final properties of the optical fibers. For example, early glass fibers had heavy optical losses due to hydroxide ions, that would not have made optical communication economically feasible. Now fibers with losses less than 0.2 decibels per kilometer are easily achieved.
Amplification by Rare Earth Elements
Rare earth elements are uniquely suited for amplification because of their relatively isolated 4f electron orbitals. Normal elements usually have the electrons in the outermost shell coupled to the neighboring atoms in the lattice. This can cause certain electronic transitions to be quickly dissipated into the lattice as heat. However, in rare earth elements, the outer 6s and 5d orbitals shield the inner 4f electrons from coupling to the neighboring lattice atoms. As a result, the electrons in 4f orbitals have higher lifetimes and take longer to decay into lower energy levels. Higher lifetimes imply better efficiency for energy drawn during light amplification.
The most commonly used rare-earth elements for amplification are neodymium, ytterbium, erbium, and thulium. For fiber amplifiers, these materials are usually excited with laser light around 800 to 900 nm from a typical GaAs based diode lasers. Then the energy from the excited state is extracted by photons around 1500 nm. Thus fiber amplifiers are the most efficient in the infrared region for amplification. The energy levels pertaining to fiber amplification in Neodymium fiber amplifiers are shown below.
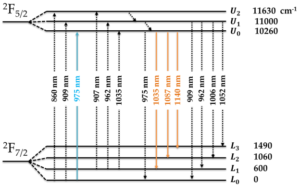
Energy levels in Neodymium: Blue for excitation and orange for stimulated emission. Courtesy InTechOpen.
The fiber amplification process is as follows: laser light is passed through the fiber to excite the dopant ions. Due to relatively high lifetimes, the dopant ions do not lose this absorbed energy easily. The light passing in the fiber of a different wavelength pertaining to correct emission energy picks up this energy as it passes through. Thus photons in the emission wavelength are increased in number.
Improving Amplification by Sensitization
Although, laser diodes exist that can excite the correct transitions in rare-earth elements, these processes are not as efficient in some elements. For example, Erbium is not efficient in absorbing incoming energy. In this case, the excitation can be made easier by using a sensitizer element such as Ytterbium. The function of the sensitizer element is to be able to absorb the incoming light more efficiently, and transfer that energy more easily onto the main dopant. Ytterbium absorbs energy at 980 nm for which InGaAs based laser diodes exist. Then the energy is efficiently transferred to Erbium dopants, and the emission can be made to happen around 1550 nm. The image below shows the different participating energy levels in Ytterbium and Erbium.
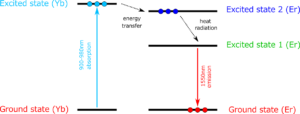
Improving efficiency by Sensitization: Erbium with Ytterbium sensitization. Courtesy FiberLabs.
This crucial discovery is what made long distance fiber optic communication economically feasible. Additionally, it enabled the development of broadband fiber amplifiers, given that there is an entire band of energy levels that the excited Erbium atoms can decay into.
Erbium Doped Fiber Amplifiers (EDFA)
EDFAs are one of the most common wide-band fiber amplifier solutions in the infrared region. Because of the sensitization effect described above, EDFAs can have low losses and good energy conversion efficiencies. Also because of the lower energy levels of Erbium being spread out, a range of output frequencies are available. These two factors have made EDFAs very popular for optical signal processing in the infrared region.
For example, digital modulation with information encoded in different frequency bands in the infrared region can be implemented using optical fibers along with EDFAs as repeaters. At the receiving end, clock and data recovery circuits are used to detect the clock rate of the information being sent, and decode the digital information. By marrying optical fiber design with optoelectronic circuitry, one can set up high bandwidth, high speed communication links not matched by any other electrical networks in terms of performance.
Properties of Amplified Light in Fiber Amplifiers
Amplification in fibers can be used to increase the output power of the fiber to several watts and kilowatts. But certain factors make it harder to increase the output power beyond that. For example, even though Yb-Er amplifiers have relatively high efficiencies, this is only about 35%, with the rest being dissipated as heat. Moreover, at high powers, non-linear effects occur that cause light to be taken away into higher wavelengths.
However, depending on the application, this limitation can be seen as a boon or a bane. For non-linear light generation, fiber amplifiers are excellent candidates. Here, the high intensities that the fiber amplifiers bring can be used to double the input frequency to produce light in the visible region. Frequency can also be varied non-linearly to make Raman Amplifiers. Check out a related FindLight article which describes Raman amplifiers and their applications in detail. Despite all this, different glass materials, such as ZBLAN glass fibers, that have lower transmission losses in the visible region are currently being investigated.
Apart from the power and frequency of the amplified output light, the output can be modified to produce pulses. Fibers are superior in this regard in producing picosecond and femtosecond pulses. Here the fiber amplifier is used along with mode-locking components, or gratings to get short pulses. For more on this interesting phenomena check out a related FindLight article on ultrafast lasers.
Did you know FindLight marketplace has a vast collection of Fiber Amplifiers, Fiber lasers, Raman lasers, and fiber components for bending and amplifying light with your setups!