Gas lasers, a cornerstone in optical engineering, find utility in diverse fields from telecommunications to medical diagnostics. This article delves into the intricacies of gas lasers, detailing the foundational physics and mechanisms that enable their function. Central to a gas laser’s operation is the gaseous medium, which undergoes external energy application to achieve a population inversion—a crucial step for lasing action. This exploration will encompass the electrical excitation processes, the vital cooling systems, and the challenges of gas regeneration.
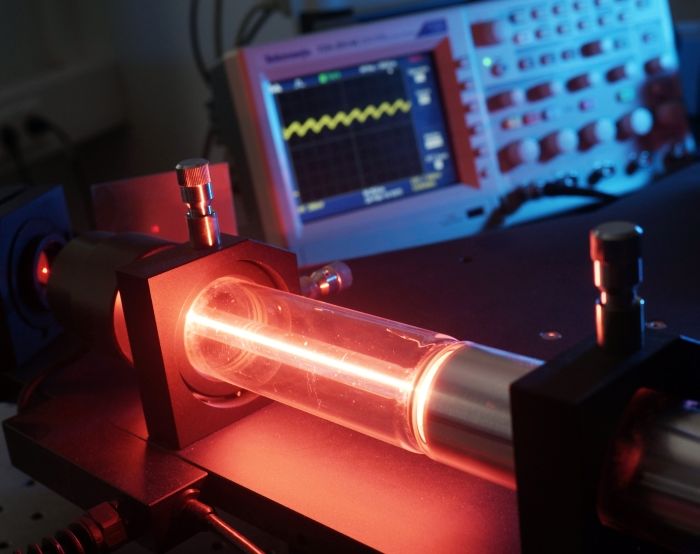
Experimental setup in an internship laboratory at the Abbe School of Photonics in Jena, taken on February 20, 2014. Photo: Jan-Peter Kasper/FSU
As we progress, we’ll dissect the various types of gas lasers, from the high-power CO₂ variants to the specialized Raman lasers. We aim to offer a clear, in-depth understanding of gas laser technology, focusing on the precision engineering and scientific principles that underpin its significance.
Today's post is sponsored by LECC Technology - a pioneer in diode laser technologies for over 20+ years.
In this Article we will cover the following topics:
- Introduction
- Fundamentals of Gas Lasers
- Historical Context
- Types of Gas Lasers
- Principles of Operation of Gas Lasers
- Applications and Modern Uses
- Advantages and Limitations of Gas Lasers
- Future of Gas Lasers
- Conclusion
1. Introduction
In the vast landscape of laser technology, gas lasers stand out for their distinctive operational mechanisms and unique applications. Originating from the mid-20th century, they have carved a niche in the annals of optical engineering, offering a diverse spectrum of output wavelengths and power scales.
Gas lasers operate by utilizing specific gas or mixtures of gases confined within a tube, which, when excited, emit coherent light. This mechanism is primarily driven by an electric discharge that produces a population inversion—a crucial prerequisite for laser action. While they might have faced stiff competition from laser diodes and solid-state lasers in recent times, gas lasers retain an irreplaceable position in several specialized fields, especially in material processing and scientific research.
In this comprehensive guide, we delve deep into the underpinnings of gas lasers, exploring their various types, principles of operation, and applications. Whether it’s the iconic helium-neon laser or the high-powered carbon dioxide laser, each has its own tale of engineering brilliance to narrate. Buckle up for a scientific journey into the heart of gas lasers.
2. Fundamentals of Gas Lasers
In the realm of photonics, gas lasers have carved a distinctive niche. Unlike solid or liquid lasers, the lasing medium in a gas laser is gaseous, and this unique characteristic grants it both advantages and challenges. Before diving deep into the principles of their operation, it’s essential to establish a foundational understanding of their core components and basic concepts.
Lasing Medium: At the heart of any laser lies its lasing medium. In gas lasers, this medium is composed of atoms or molecules in a gaseous state. The choice of gas or a mix of gases determines many of the laser’s properties, including its emitted wavelength and operational efficiency.
Population Inversion: A foundational requirement for lasing, population inversion refers to a condition where a majority of the particles in the medium are in an excited state rather than in their base state. In gas lasers, this is typically achieved by introducing external energy, setting the stage for the amplification of light.
Optical Feedback Mechanism: The generation of a coherent beam necessitates an optical feedback mechanism. Gas lasers employ two mirrors, with one allowing a fraction of light to pass through. This setup ensures the continuous amplification of light within the cavity.
Excitation Mechanisms: While we’ll delve deeper into this in the subsequent section, it’s worth noting that gas lasers employ a variety of mechanisms to excite their medium. Most commonly, this involves the use of an electric discharge.
Cooling and Regeneration: Given the high energy operations, maintaining an optimal temperature within the laser system is crucial. Moreover, the integrity of the gas medium might degrade over time, leading to the need for its regeneration or replacement.
Gas lasers, with their unique medium and fundamental characteristics, have ushered in myriad applications in both research and industry. With this foundational knowledge in place, we’ll further explore the intricate principles governing their operation in the sections to follow.
3. Historical Context
The journey of lasers through the corridors of scientific history is marked by continuous innovation and significant milestones. At the forefront of this evolution stands the remarkable gas lasers, setting the stage for subsequent laser technologies.
Introduction of Gas Lasers in Early Times: The 1960s marked the emergence of the gas laser era, most notably symbolized by the Helium-Neon (He-Ne) laser. This pioneering laser, with its characteristic red beam, quickly became a beacon for what lasers represented in the collective consciousness. Other notable gas lasers, such as the carbon dioxide (CO2) laser, followed suit, each carving out niche applications, from medical treatments to industrial cutting.
Dominance of Excimer Lasers: Excimer lasers, which rose to prominence in the late 20th century, offered unique properties that set them apart. Harnessing the power of rare gases and halogens, these lasers produce ultraviolet light, making them ideal for applications requiring precision without the accompanying heat damage. Their unparalleled accuracy made them invaluable in eye surgeries, particularly in refractive surgeries like LASIK. Furthermore, their ability to etch fine patterns led to their adoption in semiconductor manufacturing, making them instrumental in the microelectronics revolution.
Transition to More Compact Lasers: The constant push for optimization in the laser domain soon led to the advent of more compact alternatives. Laser diodes, with their reduced footprint, brought forward increased energy efficiency and found their way into everyday electronics. Meanwhile, solid-state lasers, using solid crystalline or glassy mediums, gained traction due to their high-intensity output and adaptability to pulsed laser applications.
Tracing the evolution of gas lasers, from their inception to their dominance and eventual complementation by newer technologies, offers a lens into human ingenuity. While newer laser technologies have burgeoned and diversified applications, gas lasers, especially stalwarts like Excimer lasers, continue to be pivotal in various industries, their contributions irreplaceable in the grand tapestry of laser history.
4. Types of Gas Lasers
Gas lasers have been integral to the evolution of laser technology. Each type, with its unique characteristics and emission spectra, has its place in various applications. Here’s some insight into the different types of gas lasers:
4.1. Neutral Atom Lasers
The inception of gas lasers was heralded by neutral atom lasers, which harnessed the unique properties of noble gases and certain metals. Each type, with its distinct attributes, played an instrumental role in shaping the domain of laser technology.
Helium-Neon Lasers (He-Ne lasers): Pioneered in the early 1960s, the Helium-Neon Laser became synonymous with laser technology due to its characteristic red beam at 632.8 nm. However, He-Ne lasers can also emit at other wavelengths, such as 543.5 nm (green) and 594.1 nm (yellow), albeit with lesser efficiency. Acclaimed for their coherence and stability, they’ve been employed widely from barcode scanners to holography.
Metal Vapor Lasers: Metals, when vaporized, reveal lasing potential. The Copper Vapor Laser, for example, prominently emits at 510.6 nm (green) and 578.2 nm (yellow). Gold vapor lasers, though less common, operate around 627.8 nm. These high peak power, often pulsed lasers, are coveted in dermatological treatments, high-speed photography, and select industrial processes.
Other Noble Gas Lasers: Beyond helium and neon, other noble gases like argon, krypton, and xenon have been harnessed for lasing. Argon lasers, for instance, commonly emit at 488 nm (cyan-blue) and 514.5 nm (green), while krypton lasers produce lines across the spectrum, including 647.1 nm (red) and 568.2 nm (yellow-green). Their distinct emission properties cater to specialized areas like spectroscopy and experimental physics.
4.2. Ion Lasers
Ion lasers utilize noble gas ions as the active medium. These lasers are known for their high output power and impressive continuous wave (CW) operation. They’re recognized for their deep, vivid colors, making them popular for various applications. Let’s delve into the notable types of ion lasers.
Argon Ion Lasers: Argon ion lasers primarily emit light in the blue and green spectral ranges. They are commonly used in biomedical applications, spectroscopy, and entertainment due to their stable output and distinct colors. A typical argon ion laser might produce wavelengths at 488 nm (blue) and 514.5 nm (green), although there are several lines ranging from the ultraviolet to the infrared. Their continuous-wave output and capability to function at several watts make them suitable for tasks requiring substantial power in these spectral regions.
Krypton Ion Lasers: Krypton ion lasers are versatile in their emission, producing various wavelengths across the visible spectrum. They can emit in the red (647.1 nm), green (530.9 nm), and yellow (568.2 nm) regions, to name a few. Due to their wide range of colors, they find applications in color displays, holography, and Raman spectroscopy. While they might not be as powerful or efficient as argon lasers, their diverse color output makes them invaluable for specific tasks.
4.3. Molecular Lasers
Molecular lasers utilize molecules as their active medium and are known for their varied applications, from industrial cutting to scientific research.
Carbon Dioxide Lasers (CO2 lasers): CO2 lasers are among the most powerful and efficient continuous-wave lasers. They primarily emit infrared radiation with a typical wavelength of 10.6 μm. This makes them highly effective for material processing tasks such as cutting, engraving, and welding, especially for non-metallic materials like plastics and wood.
Carbon Monoxide Lasers (CO lasers): Carbon monoxide lasers emit in the far-infrared range. They typically operate around wavelengths such as 5.4 μm and 4.8 μm. While they aren’t as widely used as CO2 lasers, their distinct emission lines find specialized applications in research and industry.
Nitrogen Lasers: Predominantly pulsed lasers, nitrogen lasers emit ultraviolet radiation with a common wavelength of 337.1 nm. They have found a niche in high-speed photography due to their short pulse durations and are also popular among hobbyists.
Bonus Info: We found this cool video on YouTube on How to Make our Own CO2 laser tube:
4.4. Excimer Lasers
These lasers derive their name from “excited dimer.” Excimer lasers utilize noble gas halides as the lasing medium and are particularly useful for ultraviolet emissions.
Excimer lasers produce light through the excitation of a temporary molecule formed from two different atoms, typically a noble gas and a halogen. Popular for applications such as eye surgery and semiconductor lithography, these lasers typically emit wavelengths in the ultraviolet range, like 193 nm (argon fluoride) and 248 nm (krypton fluoride).
4.5. Metal Vapor Lasers
These lasers utilize metal vapors as the active medium. Their unique characteristics make them suitable for various applications from imaging to research.
Copper Vapor Lasers (CVL): CVLs emit green-yellow light with common wavelengths being around 510.6 nm (green) and 578.2 nm (yellow). They are recognized for their high repetition rate and pulsed operation, making them ideal for applications like dermatological treatments and imaging.
Gold Vapor Lasers: Though not as common as other metal vapor lasers, gold vapor lasers emit at wavelengths like 627.8 nm (red). They have specialized uses in scientific research due to their distinct emission properties.
Helium-Cadmium Lasers (HeCd): Helium-cadmium lasers predominantly emit in the blue-ultraviolet spectrum. Typical emission wavelengths include 442 nm (blue) and 325 nm (ultraviolet). These lasers find applications in fluorescence microscopy and UV lithography.
4.6. Hydrogen Lasers
These lasers utilize hydrogen gas as the active medium and often operate based on the Raman effect.
Hydrogen Raman Laser: The unique feature of the hydrogen Raman laser is its ability to shift the wavelength of incident light. It is particularly effective at converting high-power visible lasers into the ultraviolet range. While not the most common type of gas laser, they have specific industrial and research applications due to this wavelength-shifting ability.
4.7. Chemical Lasers
These lasers derive their energy from chemical reactions, often involving the combustion of specific gases.
Hydrogen Fluoride (HF) Laser: Operating in the infrared region, HF lasers can emit at various wavelengths, ranging from 2.7 to 2.9 μm. These lasers are especially famous for their high-energy outputs, making them suitable for defense and research applications.
Deuterium Fluoride (DF) Laser: Like the HF laser, DF lasers operate in the infrared range. They have emission wavelengths around 3.5 to 4.0 μm. Due to their powerful emission capabilities, they find applications in military settings and scientific experiments.
4.8. Far-Infrared (FIR) and Submillimeter Lasers
These lasers operate in the far-infrared spectrum, making them useful for specialized research, particularly in molecular spectroscopy. Due to their unique operational range, they’re vital tools in studying rotational transitions in molecules. Typical emission wavelengths for these lasers range from 30 μm to 1 mm.
5. Principles of Operation of Gas Lasers
Understanding the operation of gas lasers requires a comprehension of their excitation mechanisms, cooling methods, and the techniques employed for gas regeneration. Each of these aspects ensures that the laser functions efficiently and sustains its performance over prolonged periods.
5.1. Excitation Mechanisms
Gas lasers rely on various excitation methods to achieve a population inversion, a necessary condition for lasing.
Electric Discharge Excitation: A prevalent mechanism, electric discharge excitation involves passing an electrical current through the laser medium. This can be achieved using either direct current (DC) fields or radiofrequency (RF) excitation. While DC fields are simpler and more common, RF excitation offers advantages in uniformity and longevity, particularly in situations where the electrode degradation needs to be minimized. The nature and characteristics of electric discharges can vary depending on the type of gas and the laser’s specific design.
Gas Dynamic Excitation: This method is prominently associated with gas dynamic CO2 lasers. Here, combustion processes create a rapid expansion of gases, leading to cooling and, subsequently, a population inversion. The inherent dynamics of this process are vital for the lasing action in these types of lasers.
Other Excitation Mechanisms: Several other excitation means exist, though they may not be as widely adopted as the aforementioned methods. These include excitation by electron beams, specific chemical reactions, and optical pumping. Raman lasers, for instance, employ a different principle altogether, where one photon is used to excite a molecule to a higher vibrational state, and upon relaxation, a photon with a shifted wavelength is emitted.
5.2. Cooling Mechanisms
Due to the energy-intensive processes involved, efficient cooling is imperative for gas lasers to prevent overheating and ensure consistent performance.
Heat Diffusion to Tube Walls: In many gas lasers, helium acts as a buffer gas, facilitating rapid heat dissipation from the lasing medium to the tube walls. The tube walls, usually maintained at cooler temperatures, act as sinks, drawing away the excess heat.
Rapid Gas Circulation: Some powerful gas lasers employ this method, wherein the gas mixture is rapidly circulated through a closed system coupled with an external heat exchanger. This constant circulation aids in dissipating heat away from the laser’s active region, ensuring steady operation.
5.3. Gas Regeneration
Maintaining the purity and composition of the laser gas is pivotal for its performance.
Reasons for Gas Deterioration: Over time and with continued operation, the laser gas can undergo deterioration for a variety of reasons.
- Contamination: This might arise from external sources that compromise the purity of the gas medium.
- Electrode Sputtering: Sputtering from electrodes can introduce impurities into the gas.
- Chemical Processes: Certain chemical interactions can degrade the quality or change the composition of the gas medium. For example, in CO2 lasers, there might be a transformation of CO2 into CO and O2, which can affect performance.
- Leaks: Over time, even the most robustly constructed laser chambers can develop minute leaks. These leaks might admit external contaminants or simply reduce the pressure inside the chamber, both of which can impact laser performance.
Methods to counteract these issues involve regeneration strategies that either periodically exchange the deteriorated gas or employ sealed systems with additional measures to ensure gas purity and optimal functioning.
Methods of Regeneration: Regeneration strategies involve either periodically exchanging the laser gas after a certain number of operational hours or employing sealed systems. Sealed systems typically use catalysts to combat contaminants. They also add supplementary gases. Both strategies help counter harmful chemical processes, extending the laser medium’s lifespan and performance.
6. Applications and Modern Uses
Gas lasers, owing to their reliability, tunability, and distinct lasing properties, have found a plethora of applications in diverse domains. Hence, let’s look into some key applications:
Medicine: In the world of medicine, CO2 lasers have been transformative. They offer unparalleled precision, enabling the meticulous removal of moles and warts. Furthermore, excimer lasers have become synonymous with vision correction, with procedures like LASIK allowing millions to see without the aid of glasses or contact lenses.
Material Processing: The industrial landscape has also greatly benefited from the prowess of gas lasers. The CO2 laser, with its high-power output, stands as a stalwart in metal cutting and welding applications. Beyond structural applications, it has found use in surface treatments, tailoring material properties to imbue them with qualities like enhanced corrosion resistance.
Telecommunications: Telecommunications once leaned heavily on the stable light of helium-neon lasers, especially in the infancy of fiber optics. While newer technologies have supplanted them in many communication applications, their legacy in this field is undeniable.
Semiconductor Manufacturing: The intricate domain of semiconductor manufacturing is underpinned by the capabilities of excimer lasers. In the realm of photolithography, these lasers, with their ultraviolet emissions, craft minuscule patterns, laying the groundwork for the electronics we rely on daily.
7. Advantages and Limitations of Gas Lasers
In the vast spectrum of laser technologies, gas lasers have carved a unique niche for themselves. Their journey, marked by consistent innovations, has seen them transform industries and impact numerous applications. Yet, while they exhibit distinct strengths, they also come with inherent challenges. Let’s delve into the principal advantages and drawbacks of these light sources.
Advantages: Gas lasers boast a number of distinct advantages that set them apart in the realm of photonics. Foremost is their high power output, a characteristic particularly pronounced in CO₂ lasers, enabling them to efficiently handle tasks like cutting and engraving even the hardest materials. This powerful performance is complemented by the sheer variety of wavelengths they can emit, offering a tailored solution for a plethora of applications, from medical to industrial. Furthermore, the beam quality delivered by gas lasers is often unparalleled, a trait that becomes pivotal when precision is paramount.
Limitations: However, like all technologies, gas lasers come with their set of challenges. Their complexity and maintenance requirements stand out, as they necessitate meticulous alignment, calibration, and regular upkeep. This often contrasts sharply with the simplicity of diode or solid-state lasers. Moreover, their size and power consumption can be a limiting factor, especially when energy efficiency or portability is a key consideration. Lastly, and importantly, the safety concerns surrounding gas lasers cannot be understated. Their high power outputs and the nature of the gases involved call for rigorous safety protocols, including the use of protective gear and proper ventilation.
8. Future of Gas Lasers
The horizon of technology is constantly evolving, and the world of gas lasers is no exception. While gas lasers have enjoyed decades of dominance in various sectors, they now coexist with newer laser technologies. However, the narrative isn’t about their obsolescence but about their adaptability and potential evolution.
Integration with Emerging Technologies: With the rise of quantum computing, nanotechnology, and advanced material sciences, gas lasers may find their niche in new applications. Their ability to emit precise wavelengths and maintain stability can be harnessed in tandem with the latest technological breakthroughs. For instance, as quantum systems require highly specific energies to transition quantum states, gas lasers could play an essential role in future quantum technology developments.
Improved Efficiency and Miniaturization: As in many areas of technology, the drive towards miniaturization and higher efficiency continues to push the boundaries of what’s possible. Gas lasers, traditionally bulkier than their solid-state counterparts, could witness a transformation towards compact designs without compromising their output power or stability.
Eco-friendly and Sustainable Designs: As global emphasis shifts towards sustainability, the gas laser industry might see a trend towards eco-friendly gases, recyclable materials, and energy-efficient systems. This not only aligns with global sustainability goals but also ensures the longevity and acceptability of these lasers in various industries.
Customized Gas Lasers for Niche Applications: With advancements in research, we may see the development of gas lasers tailored for highly specific applications. Be it in the medical field for unique therapeutic techniques, or in industrial applications for specialized cutting or engraving, the customization potential is vast.
Collaborative Systems: The future may not be about one laser type dominating the others. Instead, it might be about how different laser systems can work in tandem. Hybrid systems combining the strengths of gas lasers with other laser types could offer unparalleled performance, covering the entire spectrum from UV to far-infrared.
To sum up, while the landscape of laser technology will undeniably continue to diversify and expand, gas lasers are poised to adapt and thrive. Their inherent advantages, coupled with continuous innovations, ensure that they remain significant contributors to the laser community’s future endeavors.
9. Conclusion
Navigating the intricate world of gas lasers reveals a dynamic journey from their conception to the diverse range of applications they serve today. Their unique characteristics have not only paved the way for numerous technological advancements but have also persisted amid the rise of newer laser types. While they might seem like relics of the past to some, their resilience, adaptability, and continuous development suggest otherwise.
The in-depth exploration of their fundamentals, coupled with a keen understanding of their advantages, limitations, and potential future trajectories, paints a comprehensive picture. It’s evident that gas lasers, with their distinct benefits and versatile applications, are far from fading into the annals of history. Instead, they continue to evolve, finding relevance in novel areas and merging seamlessly with emerging technologies.
In the grand tapestry of photonics, gas lasers undoubtedly hold a prominent place. Their legacy, marked by a blend of historic milestones and promising innovations, serves as a testament to their enduring impact on science and industry. As we look ahead to the future of laser technology, a few things stand clear. Gas lasers will persistently illuminate our path. They will shed light on new territories and open doors to possibilities we’ve yet to explore.
Further Reading: Gas Lasers Explained