An object that has a temperature greater than absolute zero emits some type of radiation. More often than not, objects surrounding us emit radiation in the form of heat that is not visible to the naked eye. This is because radiative heat is in the infrared range of the light’s spectrum. The infrared spectrum can itself be divided into three distinct categories: near-infrared or NIR (wavelength: 0.75 – 1.4 micrometers), short wavelength infrared or SWIR (wavelength: 1.4 – 3 micrometers), mid wavelength infrared or MWIR (wavelength: 3 – 8 micrometers), long wavelength infrared or LWIR (8 – 15 micrometers), and far-infrared or FIR (wavelength: 15 – 1000 micrometers).
Recalling that infrared light is part of the electromagnetic spectrum that is outside the visible light portion, special instruments are needed to detect light at these wavelengths. One such instrument is called a passive infrared sensor. The reason why this type of infrared sensor is referred to as being passive is because it detects infrared light without generating or radiating energy.
The type of infrared sensor that emits infrared radiation that is later reflected from the object and received by the receiver is called an active infrared sensor. More specifically, active infrared sensors emit infrared wavelength via an IR Light Emitting Diode (LED). After reflecting from an object this radiation is later collected/received by either the photodiode, phototransistor, or a photoelectric cells.
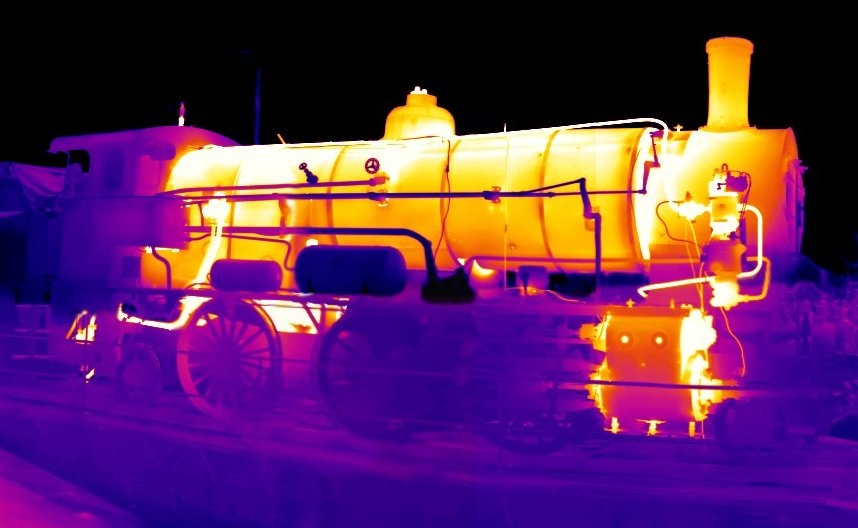
This image was produced by detecting the heat signature from the source. Thermal IR sensor was used to produce this image. The red/white areas in the image represent portions of the source that is emitting high thermal radiation. Courtesy of Google Images.
Thermal vs Quantum Well Infrared Sensor
Generally IR sensors can be grouped into two categories: Thermal IR sensors and Quantum well IR sensors. As the name suggests, thermal IR sensors use infrared energy as heat for detection. The sensitivity of this detector is independent of wavelengths, therefore, it is capable of detecting objects in the near IR, mid IR, and far IR bands. In addition, these detectors do not require cooling after exposure, however, they do suffer with slow response times and low resolution.
In contrast to thermal detectors, quantum well detectors can only detect objects at a given IR range (3 – 20 μm). This corresponds to objects in the mid and far IR bands. A quantum well photodetector exploits the electronic inter-sub-band transitions in quantum wells to absorb photons. Typically made of GaAs, these detectors operate at specific wavelengths corresponding to the discrete energy states within the quantum well. These detectors can provide higher detection performance. For example, since these detectors focus at a specified wavelength range, the resolution of the images is much greater in comparison to the images obtained from thermal detectors.
Microbolometer-Based Infrared Camera System
One of the thermal IR detectors that is currently being used for infrared imaging is called the Microbolometer-based IR camera. They operate by measuring the resistance change of the active elements within the camera upon being heated due to absorption of IR radiation. Even though this particular camera does not have the best sensitivity, it covers a good range of the IR spectrum (7.5–14 μm) which is enough for detecting objects at very low light. These cameras are less costly relative to other IR cameras. The main reason why this camera is inexpensive is because it does not have an active cooling mechanism built in. On the flip side, the images produced by this camera do not possess the most accurate measurements.
One way to numerically visualize how insensitive uncooled Microbolometers are in comparison to cooled IR cameras is by measuring their respective noise-equivalent temperature difference (NETD) values. NETD essentially provides the detector’s ability to distinguish the internal noise of the detector from the IR images. Therefore, the higher the resolution, the smaller the NETD value. For example, cooled IR cameras that are photon based have NETD values less than 20 mK whereas Microbolometers have NETD values around 50 mK.
We can find Microbolometers in our cars. The ‘collision prevent system’ uses microbolometers to detect thermal sources around the car that may result in a crash. In addition, some cars have microbolometers installed in the windshield to activate windshield wipers during rain and snow.
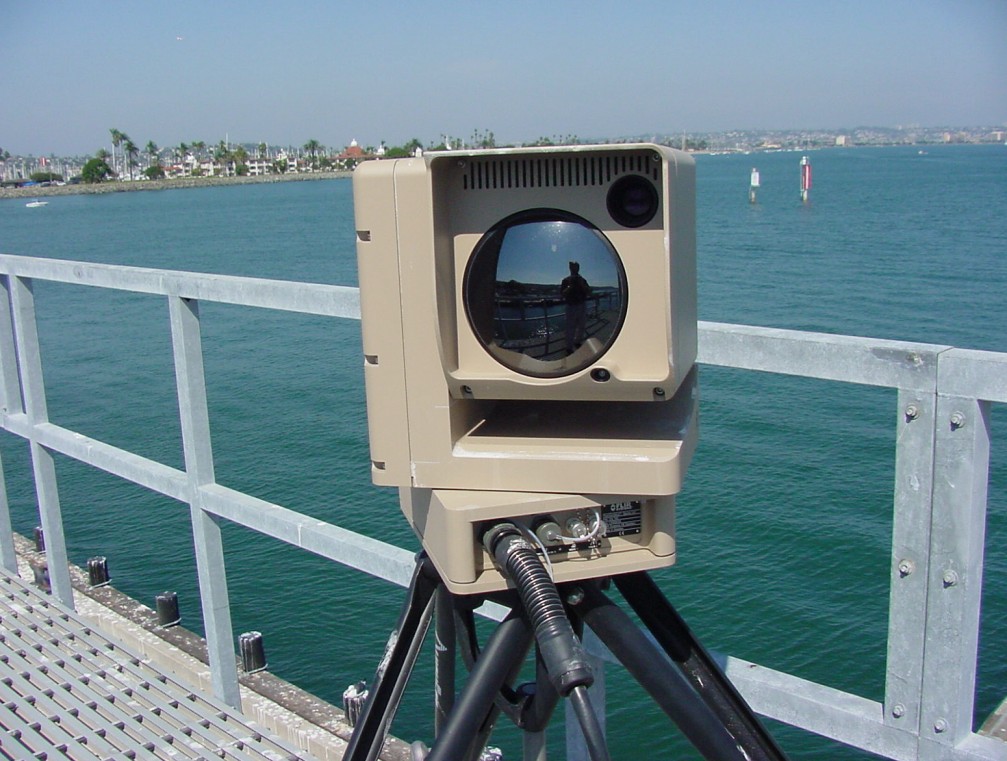
A microbolometer-based IR camera. Even though the resolution of these cameras is not the best due the lack of a cooling system, their low price make them an attractive choice among many photographers. Courtesy of Google Images.
HgCdTe Cameras
Another type of infrared camera is known as the Mercury Cadmium Telluride (HgCdTe) camera. HgCdTe is the only type of material that can detect infrared radiation in two atmospheric windows: the mid-wave infrared window (MWIR, 3 – 5 micrometers) and the long-wave window (LWIR, 8 – 12 micrometers). Depending on the cooling, those cameras can also reach the SWIR spectral range (1-2.9um). HgCdTe cameras that detect in the LWIR range need very powerful cooling mechanisms (T = 77K) to reduce noise caused by thermally excited current carriers. On the other hand, MWIR HgCdTe cameras can operate at much higher temperatures but their performance is not as good as the performance of LWIR HgCdTe cameras.
When comparing HgCdTe cameras with Microbolometers, one main difference that stands out is the cost. Sophisticated cooling systems installed in the HgCdTe cameras make them heavier and significantly more expensive relative to Microbolometers. Moreover, HgCdTe cameras require regular maintenance for peak performance.
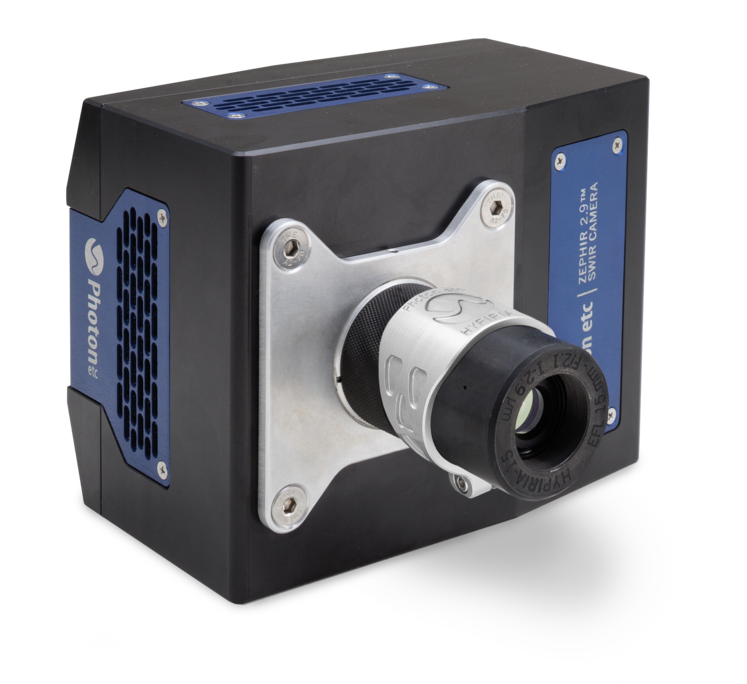
This image portrays what an operable HgCdTe IR camera looks like. Even though this camera appears like any other optical camera, its built-in cooling mechanism makes it one of the best IR cameras out there. Courtesy of Photon Etc. Click here for more information about this camera.
InGaAs Cameras
Indium gallium arsenide (InGaAs) is an alloy. One of its main applications is as an infrared detector. Photodiodes that are made up of InGaAs operate in a preferred wavelength range of 1.1 µm < λ < 1.7 µm. When compared to photodiodes made up of other elements, such as Germanium (Ge), InGaAs photodiodes have higher quantum efficiency, faster response time, and lower dark current for the same sensor area. Today, many universities are heavily investing in InGaAs cameras because their higher quantum efficiency is key in capturing very high quality infrared images, especially in the Near Infrared (NIR) and Shortwave Infrared (SWIR) in the spectrum, for scientific research. An excellent example of InGaAs camera can be found here.
Ge Infrared Sensor
It is true that photodiodes made from Germanium are not as efficient and sensitive when compared to InGaAs photodiodes, however, their properties allow them to be excellent IR sensors. Ge is a very hard, high-density material that allows IR from 2µm but blocks ultraviolet wavelengths. In addition, Ge has the highest refractive index when compared to any other IR transmitters available in the market and has a low optical dispersion.
Summarizing Infrared Sensors
Similar to our eyes that can detect wavelengths in the visible spectrum, infrared sensors detect wavelengths in the infrared spectrum. For scientists and other professionals who do infrared photography, it is crucial for them to choose the IR camera that best suits their needs. For example, someone who sacrifices the resolution of IR images for affordability, he/she would most likely prefer using a microbolometer camera. However, if someone prefers taking IR images in either the LWIR or MWIR range, he/she would use a HgCdTe. The main purpose of this article was to give a brief overview of infrared sensor and types of cameras available for infrared imaging. However, in a future article, we will go over various applications associated to infrared sensors.
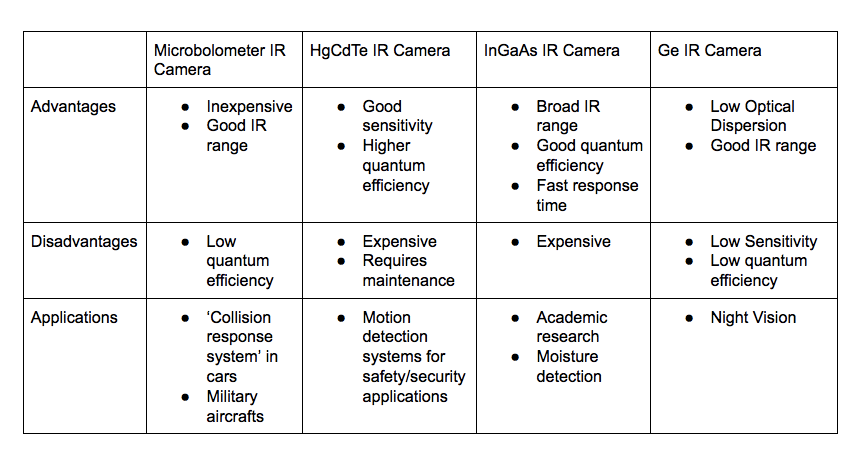
This table summarizes some of the key IR cameras covered in this article. Along with the applications, pros and cons are provided for each camera, serving as a guide for future IR camera buyers.
Further Reading
- HgCdTe Camera – Wikipedia, https://en.wikipedia.org/wiki/Mercury_cadmium_telluride
- Microbolometer-based infrared camera – Wikipedia, https://en.wikipedia.org/wiki/Microbolometer
- Passive Infrared Sensors – Wikipedia, https://en.wikipedia.org/wiki/Passive_infrared_sensor
- InGaAs Camera – Wikipedia, https://en.wikipedia.org/wiki/Indium_gallium_arsenide