Story of the Mars mission
The introduction of low-cost fiber-optic laser amplifiers is one recent advance in photonics that has rekindled interest in directed-energy propulsion. It has now been proven at laboratory scales that it is possible to modularly phase-lock massive arrays of fiber-optic laser amplifiers together, allowing them to function as a single optical element of arbitrary enormous size and power. The laser array might be constructed on Earth rather than in space by using atmospheric compensation methods that were first employed in astronomy (i.e., adaptive optics that can successfully remove the beam aberrations brought on by Earth’s atmosphere). The application of these advancements to interstellar flying has been studied in the past. True interstellar voyages would be possible with dense laser arrays on the scale of kilometers with fluxes on the order of 1 kW/m^2 leaving the array because the photon pressure of the laser would easily accelerate 1-m-scale lightsails to 20%–30% of lightspeed. Such a lightsail could return photos from nearby exoplanets within a 25-year mission if it were pointed at nearby solar systems. In this article, we explain some aspects of the importance of the laser-thermal propulsion for Mars mission. This article is mainly based on the researches accomplished by the scientists at McGill University.
A combustion reaction is provided by laser energy and then it increases the momentum of the spacecraft more immediately for interplanetary flight. Laser-based directed energy options include laser-thermal propulsion, laser-electric propulsion, and others. The two subtypes of laser-thermal propulsion are (1) laser-thermal propulsion employing a heated and expanded gaseous propellant (usually hydrogen) and (2) laser ablation propulsion using an initially condensed-phase reaction mass. The continuous-wave characteristic of phased-array lasers using atmospheric correction lends itself well to the second strategy. The focus of the current research is on the use of this type of laser-thermal propulsion in the design of deep space missions employing a big phased-array laser.
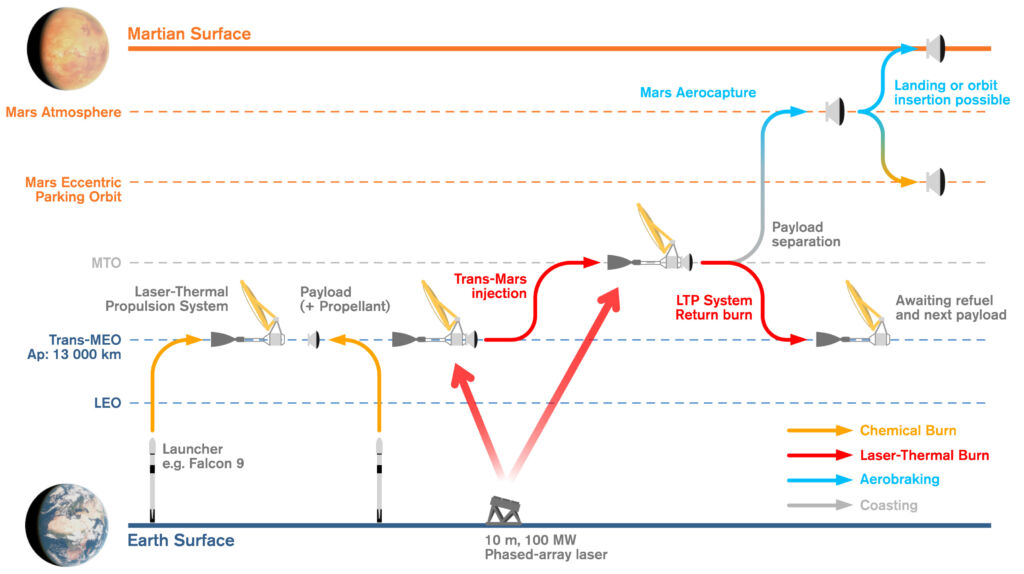
Figure 1: Operation diagram for a reusable laser-thermal propulsion system. Photo courtesy of Elsevier
Beginning in the 1970s, when the first continuously burning hot plasma supported by a laser was seen in a lab setting, laser-thermal propulsion underwent intensive research. Following this finding, Arthur Kantrowitz gave a speech in which he proposed using lasers to directly heat rocket propellant. This speech served as a catalyst for the subsequent three decades’ worth of LTP development. According to a historical account of laser propulsion research (see e.g., Fig.1 and Fig. 2) at the Marshall Space Flight Center, despite encouraging preliminary results, experimental research in this area was discontinued in the mid-1980s due to a lack of funding to maintain the sophisticated laser systems and a lack of political interest.
The challenge of “45 days”
The distance between Earth orbit and Mars orbit can be traveled in no more than 45 days, a distance of 5 AU can be traveled in no more than one year, a distance of 40 AU can be traveled in no more than five years, and a distance of 125 AU can be traveled in no more than ten years, according to a recent NASA solicitation for revolutionary propulsion for rapid, deep-space transit.
Concerns about astronaut exposure to galactic cosmic rays (GCRs) and the potential hazard of coronal mass ejections (CMEs) during transit are likely what led to the “Mars in 45 days” requirement. Recent in-situ measurements by the NASA Curiosity Rover have demonstrated that the radiation environment on Mars’ surface is two times less intense than what is felt when traveling into Earth’s magnetosphere. This conclusion shows that propulsion technologies should be prioritized together with mission concepts that shorten the transit time for crewed trips to Mars.
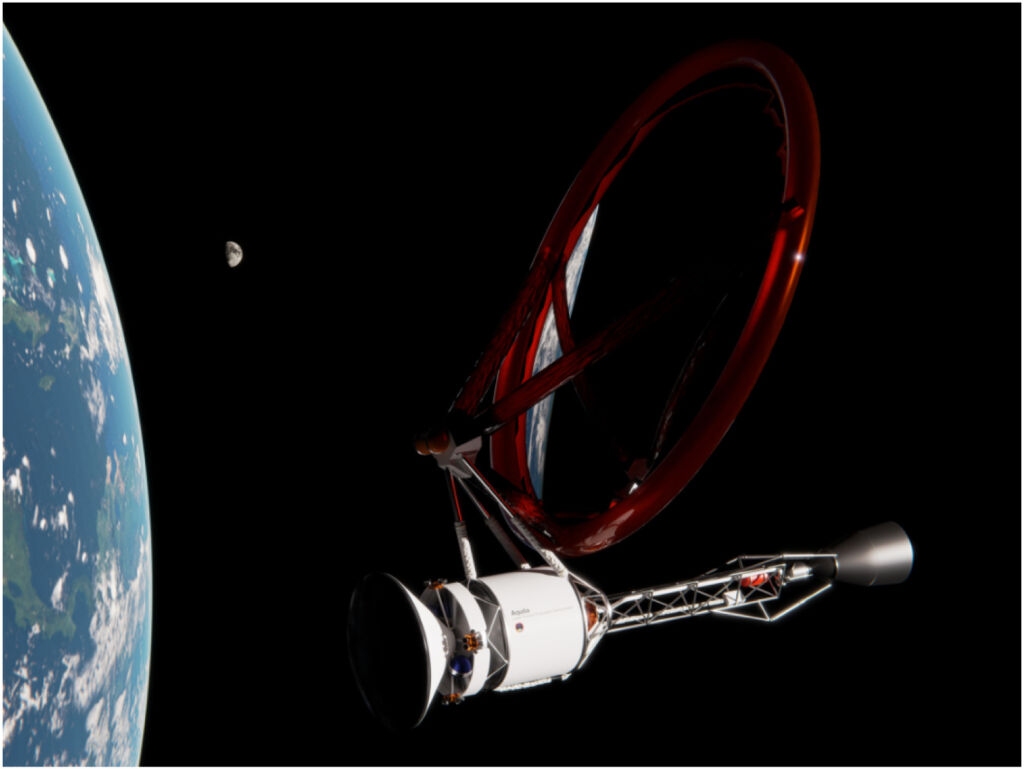
Figure 2: Render of a Laser-Thermal-Propulsion System. Photo courtesy of Elsevier
Rapid expeditions to Mars have expanded into a useful metric for contrasting various propulsion concepts, including fusion, solar electric propulsion, nuclear electric propulsion, and nuclear thermal propulsion. This is why the researchers chose a rapid-transit to Mars mission as the foundational design for their investigation.
Quick transit missions and its challenges
The consequences of deploying a 10-m-scale phased-array laser that is based on Earth and used for quick transit missions throughout the solar system, and particularly rapid transits to Mars, are examined in this research. The ability to focus the laser power delivered to the spacecraft (see Fig. 3) into a hydrogen heating chamber using large inflatable reflectors with high reflectivity and the ability to withstand intense laser flux can produce specific impulses and thrust levels (upon expansion through a nozzle) comparable to advanced gas-core nuclear thermal rockets.
The present work extensively examines the heating chamber design (see e.g., Fig. 4), which has been highlighted as a key component of the architecture. Additionally considered are the delivery method, regenerative cooling, and propellant storage. Much emphasis is also paid to innovative aerocapture techniques that are required by the high approach velocity because a laser will not be available for deceleration once on Mars. In contrast to a one-time usage scenario, the ability of the Mars-injection propulsion stage to return to Earth by executing a burn-back maneuver while still inside the focal range of the Earth-based laser is examined.
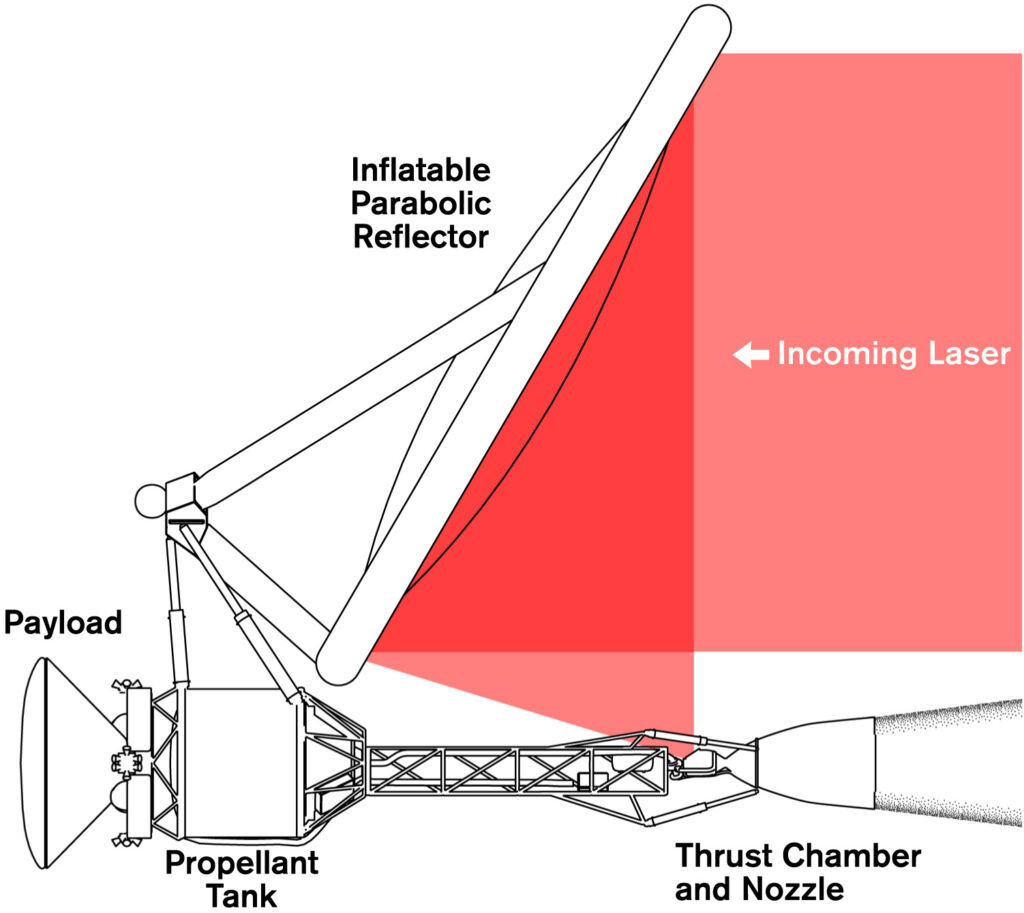
Figure 3: Spacecraft architecture. Photo courtesy of Elsevier
Architecture for Rapid Mars transit mission design
A 10-m-diameter laser array with a maximum output power of 100 MW and the ability to focus on a target up to 50 000 km away would be necessary for an LTP system for interplanetary transfers. As opposed to laser electric propulsion, which would require many arrays to maintain a steady supply of laser power, LTP maneuvers for missions evaluated in this study would last anywhere from a few minutes to an hour (depending on the mission). This proposed architecture is an appealing application to early prototype laser arrays due to the characteristic of laser-thermal propulsion, acting as a precursor to the more ambitious infrastructure (kilometer-scale arrays) needed for directed-energy interstellar travel.
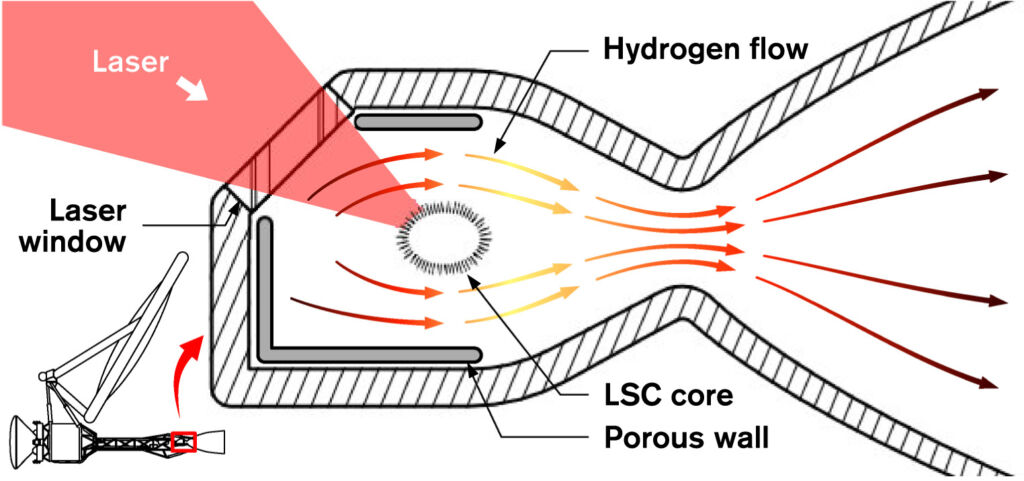
Figure 4: Laser-supported combustion wave thrust chamber schematic. Photo courtesy of Elsevier
Mission profile for Rapid Mars transit mission design
Fig. 1 displays the mission idea of operations. The launch of the laser-thermal-propulsion system (LTPS, see Fig. 5), hydrogen propellant, and payload signal the start of the mission. This can be done independently or all at once using a Falcon 9 or Atlas-class launch vehicle. A fuelled LTPS and payload are launched into an elliptical orbit with an apogee above the van Allen belts (Trans-MEO, 13000 500 km). This configuration is shown in Fig. 2. This orbit allows for the necessary dwell time above the ground-based laser, allowing the laser-powered propulsive maneuver—which lasts for about an hour—to happen during a single pass over the laser ground site.
The payload is discharged into the high-energy transfer orbit to Mars at the end of this propelling operation. In order to quickly reuse the entire LTPS gear after on-orbit propellant transfer, the LTPS undertakes a second laser-powered maneuver to bring it back to the original elliptical orbit after payload release while it is still visible and within the ground-based laser’s field of view. The payload simply needs a few small trajectory modifications to be made during the brief ballistic transfer to Mars, and then it can be aerocaptured when it gets there. Direct entrance is not thought possible because of the high approach velocity at Mars, however aerocapture can be followed by either entry and landing or by insertion into a parking orbit around Mars.
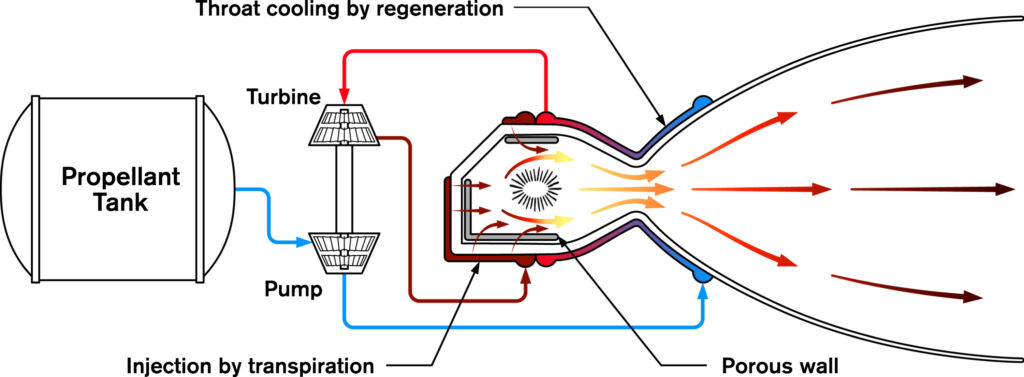
Figure 5: LTPS feed system diagram. Photo courtesy of Elsevier
Conclusions
In this article, we discussed and reviewed some recent researches conducted by the scientists at McGill University about the importance of laser-thermal propulsion for Mars missions. Here we list two important take-home messages:
- Fundamental technological barriers have not been encountered during the preliminary design of the crucial subsystems required for such a spaceship to actualize this propulsion system. Due to the fact that the power source is still on Earth and the delivered flux may be processed by a low-mass inflatable reflector, laser-thermal propulsion can attain mass-to-power ratio values that are unmatched, well below even those reported for sophisticated nuclear propulsion technologies.
- We want to emphasize that the “Mars in 45 days” objective is just used as a handy yardstick for propulsion concepts. The ability to transport personnel to the lower radiation environment on the surface of Mars while utterly limiting exposure to GCRs and probable CME events in transit is what drives this demand. This study also examined cargo delivery missions, revealing a potential ten-fold increase in payload capacity over the Common Centaur cryogenic upper stage. This architecture may make it possible to carry out other interesting missions that were previously thought to be infeasible with conventional chemical or solar-electric propulsion, such as quick missions to the outer ice giant planets, into the interstellar medium, intercepting interstellar objects traveling through the solar system, and to the solar gravitational focus.
This article is brought to you by FindLight with the support of DataRay Inc. - a leading designer and manufacturer of laser beam profile and power measurement solutions