For women across all socioeconomic groups, breast cancer poses a large threat. Approximately 1 in every 8 women will be diagnosed with the cancer during her lifetime. Additionally, it is one of the leading causes of cancer-related deaths among women. Treatment is improving, largely due to large public health efforts encouraging annual screening tests using X-ray based mammographies and MRIs. These large imaging devices are costly, and are hard to find in developing and low-resource environments. Therefore, to assist screening efforts in such communities, many groups are researching alternative detection methods. A research group from the University of Saskatchewan describes their portable and low-cost fluorometer for the detection of breast cancer in a recent paper.
Fluorescence Imaging
Fluorescence imaging is an imaging technique which uses fluorescent dyes or proteins. These dyes or proteins label specific molecular processes or structures. With fluorescence imaging, a wide range of observations can be made, from gene expression to protein expression to molecular interactions in cells and tissues.
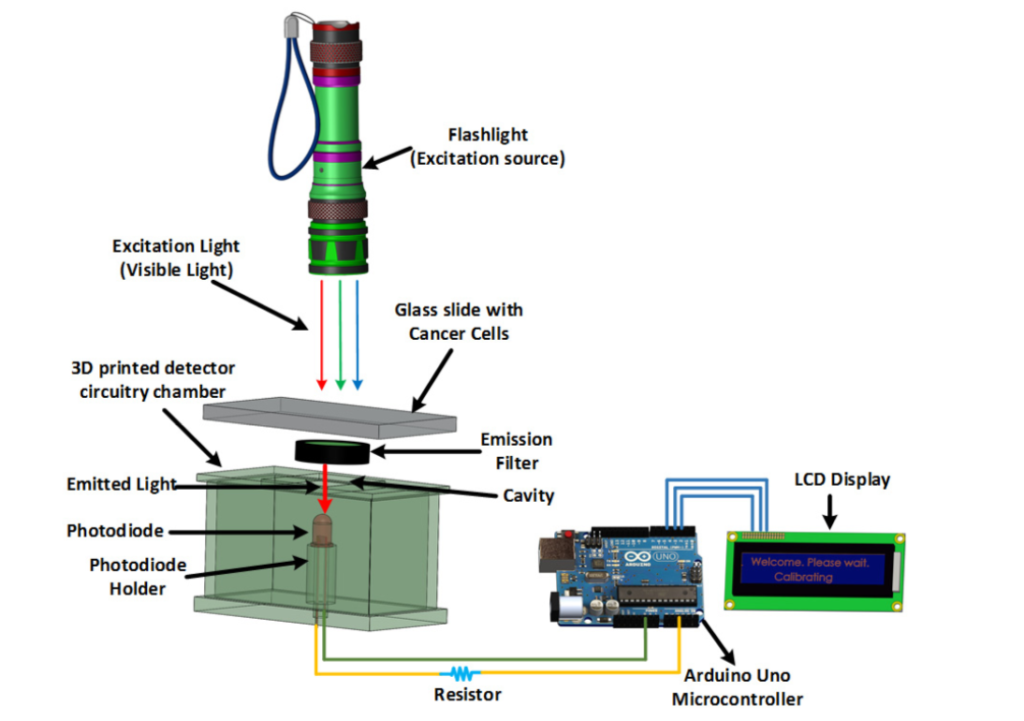
The diagram above details the various working components that would be used in this fluorometer. It differs from other fluorescent imaging-based cancer detection devices as the materials are all relatively inexpensive and easy to come by. Image courtesy of Biomedical Optics Express.
Fluorescent microscopes work in a similar way to conventional light microscopes. However, fluorescent microscopes use a higher intensity light source to illuminate samples. This illumination light is absorbed by fluorophores, which are fluorescent substances used to label specific parts of the sample, such as a specific protein. Upon absorption, the fluorophores emit light at a lower energy wavelength. Filters in the set-up only allow this emitted light to pass through, so that it can then be measured and visualized.
Fluorescence imaging techniques have already shown success regarding detection of cancerous cells in vivo. These techniques label cells of interest by using selective cancer cell-permeable fluorescent chemical/dye or a fluorophore targeting a cancer-specific protein of interest. However, current imaging devices for this purpose are expensive and rely on sophisticated hardware and software. Therefore, researchers from the University of Saskatchewan focused their efforts into developing a low-cost alternative.
The Fluorometer
The final device designed by the team, of which a preliminary diagram is shown above, is shown below. It consists of a flashlight, an Arduino Uno microcontroller to collect and transmit data, a photodiode, which detects fluorescent signals, a 3D printed sample chamber, an emission filter, and an LCD display to display results. The chosen photodiode works in the range of 400nm-1,100nm. The fluorometer does not provide a processed image of the sample like a traditional microscope would; instead, it displays a message indicating if a cancer cell was detected or not.
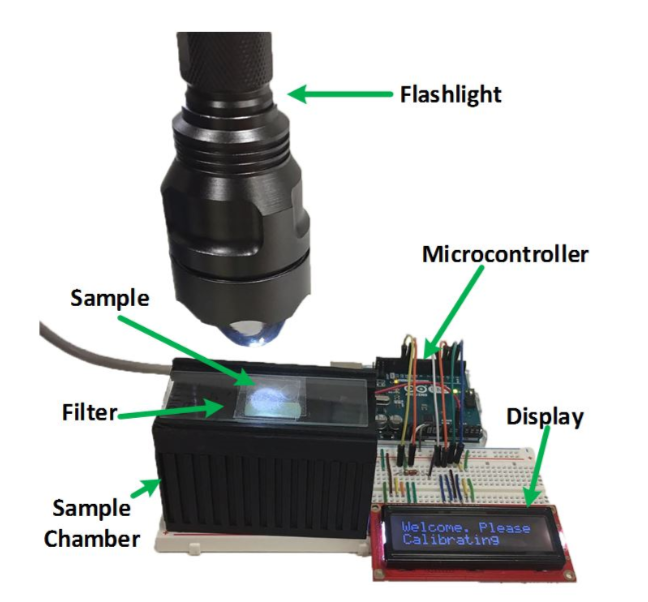
The photograph above displays the experimental set-up of the finished fluorometer. Researchers selected parts that were cheap and easy to find, so that this design could be replicated in low-resource laboratories as well. Image courtesy of Biomedical Optics Express.
A control cell sample is used to calibrate the device. The photodiode generates an analog signal which is then converted into a digital signal by the microcontroller. 10 readings are performed per sample and an average value is calculated. Next, the control cell sample is removed and an unidentified sample is placed in the sample chamber. Once again, 10 readings are performed. The average value of the unidentified cell sample is subtracted from the control cell sample’s average. If the difference is greater than 700mV, the unidentified cell is qualified as cancerous. The operating procedure is shown below.
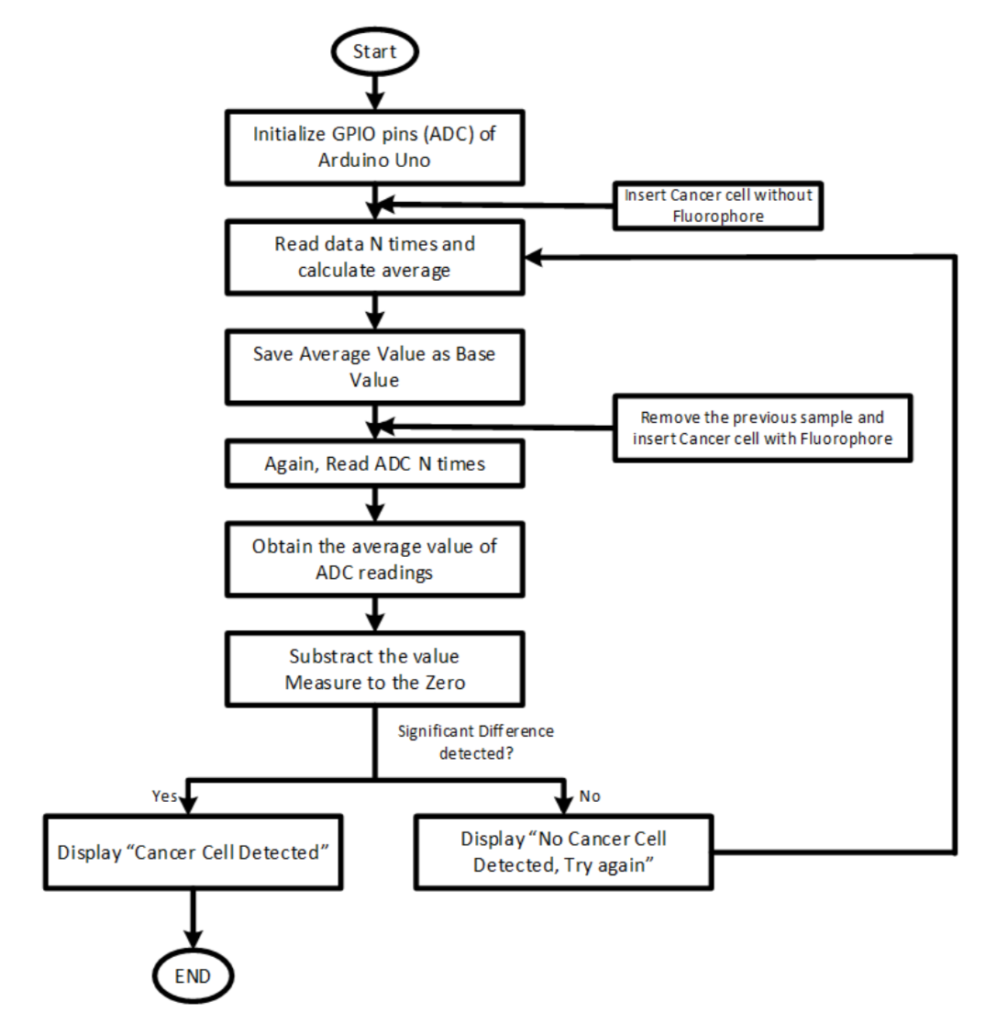
The diagram above is the operating procedure of the fluorometer. Image courtesy of Biomedical Optics Express.
Results of Initial Testing
For testing, normal and breast cancer cells were obtained and cultured in vitro. Green Fluorescent Protein (GFP) was added to the cells via retroviral transduction. Traditional microscopy imaging was used to confirm which cells were cancerous and which were not. 20 samples were used in total: 10 non cancerous, and 10 cancerous. It was noted during preliminary testing that a minimum confluency of 60% was necessary for the fluorometer to be accurate; therefore, all samples used had a confluency of 60%.
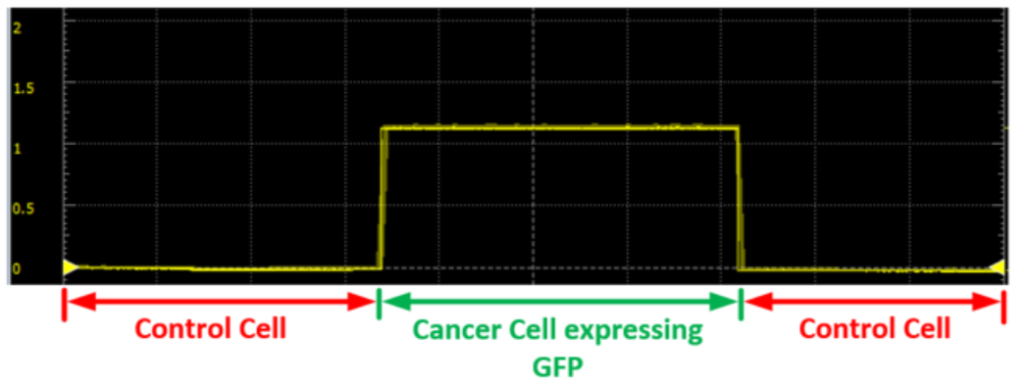
The graph above displays the readings measured on a waveform oscilloscope. As can be seen, the measured voltage is non zero for cancerous cells. With non-cancerous cells, the average value measured is close to zero. Image courtesy of Biomedical Optics Express.
The fluorometer correctly identified all of the control cells. It misidentified one cancer cell, marking it at non-cancerous. This misidentification was explained as being due to inhomogeneous confluency in the field of the coverslip of the cancerous cell.
Conclusion
The University of Saskatchewan team was satisfied with their initial results. The total cost of the device was just under $140 USD. More than fifty percent of the cost came from the purchase of the emission filter. As the manufacture of the device required cheap and accessible parts, the design would be easy to replicate in a variety of medical and research laboratories, including those in low-resource settings. As the device weighs 0.16 kg and has dimensions of 8cm x 4cm x 3.2cm, it is much more portable than other fluorescent imaging devices.
Further testing and designing would involve adapting the flashlight and testing a wider range of sample variables. For the experiment detailed, an experienced user would need to manually adjust and hold the flashlight. Further designs would include a mechanical system that holds the flashlight appropriately. Future testing on samples of varying composition or thickness would be done to test the accuracy of the fluorometer and measure its success with a greater sample range.
Additionally, the fluorometer, with slight modifications to the filter and photodiode, could be used for the detection of cancers other than breast cancer. This would be necessary as differing tumors or cancers may require different fluorophores used for identification.
To read more on this low-cost fluorometer and the team’s efforts, the paper can be viewed here.