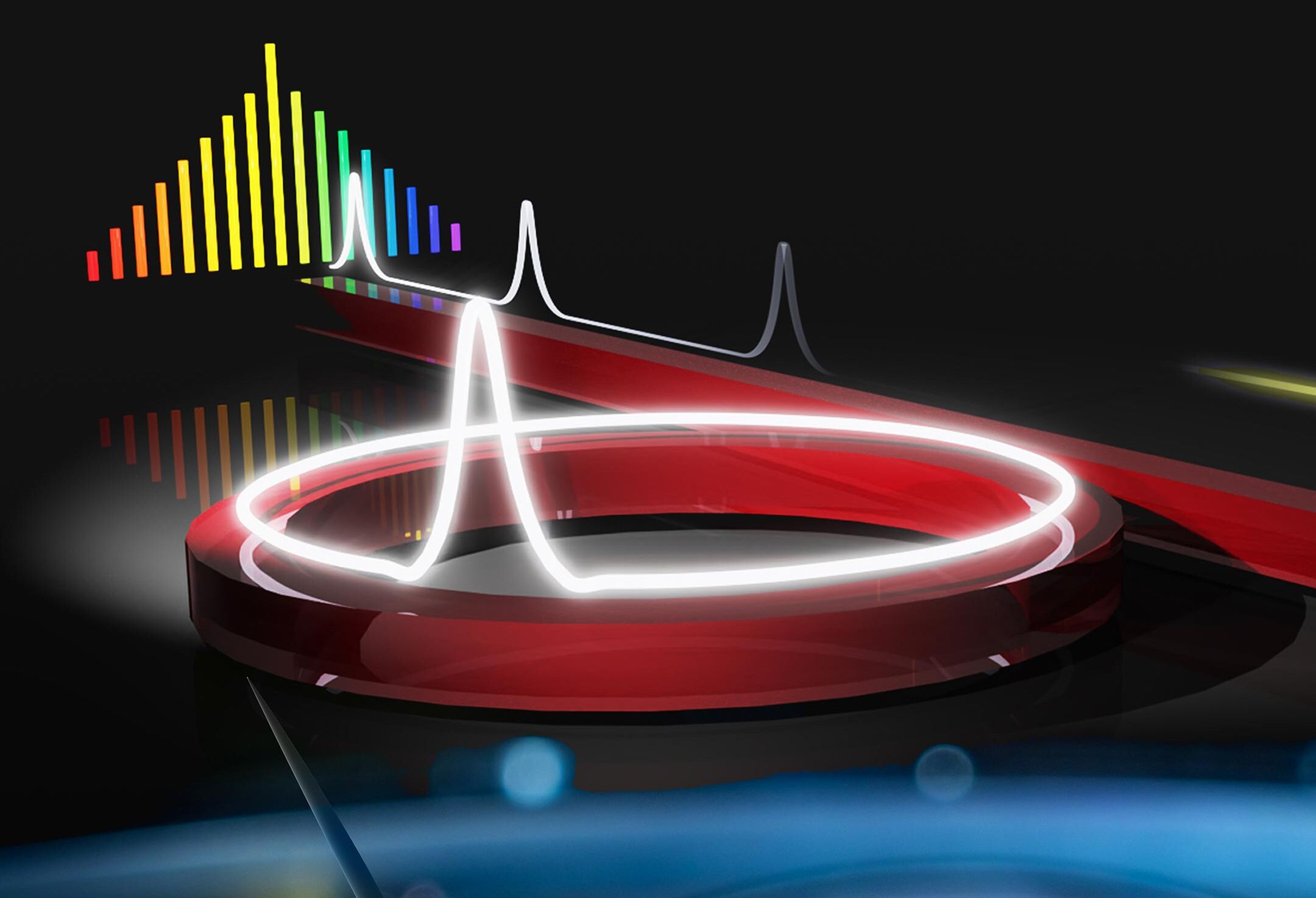
Microresonators: artistic rendering of an optical microresonator. Image Courtesy of SciTechDaily
Introduction
Microresonators have been the topic of interest for many research groups for their unique properties, compactness, ease of fabrication, and versatility. They are essentially optical cavities that couple some of the light traveling in one or more input ports into a ring or a cavity resonator where resonant wavelengths constructively interfere and then couple out of the ring into another waveguiding structure. The coupling condition depends on 3 parameters: the size of the gap separating the waveguides and the cavity resonator, the coupling length, and the refractive indices of the materials used to fabricate these components. By manipulating and optimizing these parameters, researchers developed several configurations of microresonators with applications in optical communications, quantum optics, sensing, and non-linear optics.
In this article, we will present different existing configurations of microresonators and some of their most popular applications. Here is a brief content table with links o the relevant sections:
Different Configurations of Microresonators
Before discussing the different configurations of microresonators, it is worth learning about some of their figures of merit. Perhaps one of the most important figures of merit of microresonators is the quality factor Q, also known as the Q-factor. It is used to determine the spectral range of the device as well as the amount of loss caused by scattering or bending in the resonator due to surface roughness. As a result, loss reduction results in high values of Q-factor. Another figure of merit which is directly proportional to the Q-factor is the photon lifetime or resonator lifetime. A long resonator lifetime indicates longer temporal confinement, a property desirable for sensing applications. It is also important to note that these parameters depend on the geometry and the configuration of the microresonator.
Microring / Microdisk Resonator
This is the most basic and first developed configuration of microresonators. It is comprised of a single bus or two bus waveguides coupled to a circular ring, all situated on the same plane (lateral geometry) or separated by a vertical gap (vertical geometry). An illustration of the lateral geometry is shown below.
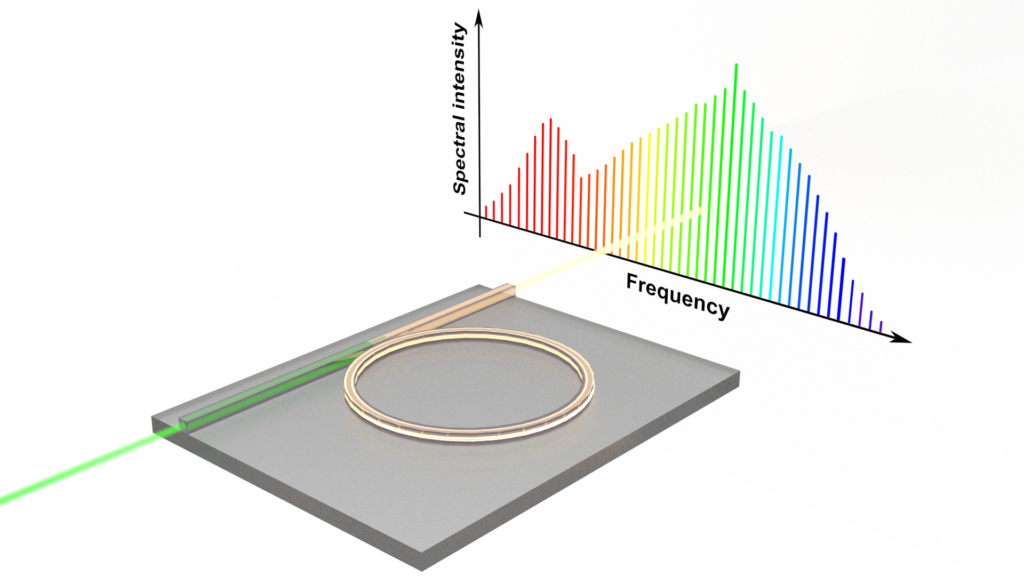
Single bus ring resonator in lateral geometry. Courtesy of Wikimedia Commons.
With this configuration, the performance of the device can be enhanced when the gap between the ring resonator and the waveguide is reduced, but such design is limited by fabrication constraints.
Pulley Type Microring Resonators
As its name suggests, the pulley type microring resonator takes the shape of a pulley where the normally straight bus waveguide wraps around a section of the ring. This configuration is characterized by a low bending loss and offers a longer coupling region (region over which the bent waveguide and the ring overlap). The latter property can significantly enhance the performance of the resonator when the phase-matching condition between the ring and the pulley waveguide is reached. In other words, when the effective index of refraction of the curved waveguide and the ring are matched, the coupling or light transmission from the pulley waveguide to the ring resonator becomes proportional to the coupling length. Pulley type microring resonators offer a higher Q-factor thanks to their low loss characteristic. The device configuration is shown below.
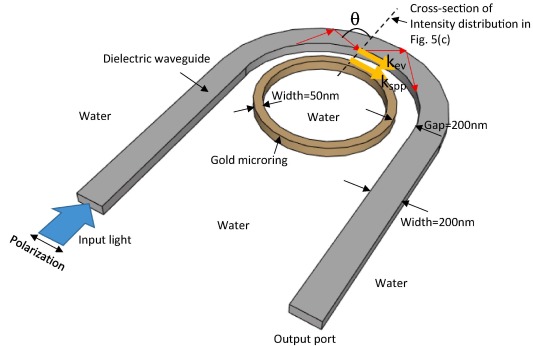
Pulley type microring resonator where light couples from the bent waveguide into the ring . Courtesy of ScienceDirect.
Racetrack Microresonators
Racetrack resonators have the same configuration as the basic microring resonator, but the circular ring is replaced with a racetrack waveguide which can be coupled to a single bus or two bus waveguides. This configuration is advantageous as the coupling region between the straight waveguides and the racetrack is long allowing for the lateral separation between the straight waveguide(s) and the racetrack to be larger. This makes it preferable to the basic microring resonator configuration as it increases the fabrication tolerances. However, this configuration suffers from losses due to the junctions between the bent portion of the racetrack and the straight waveguides as well as the bending losses. As a result, a racetrack resonator is characterized by smaller Q-factor values compared to microring resonators.
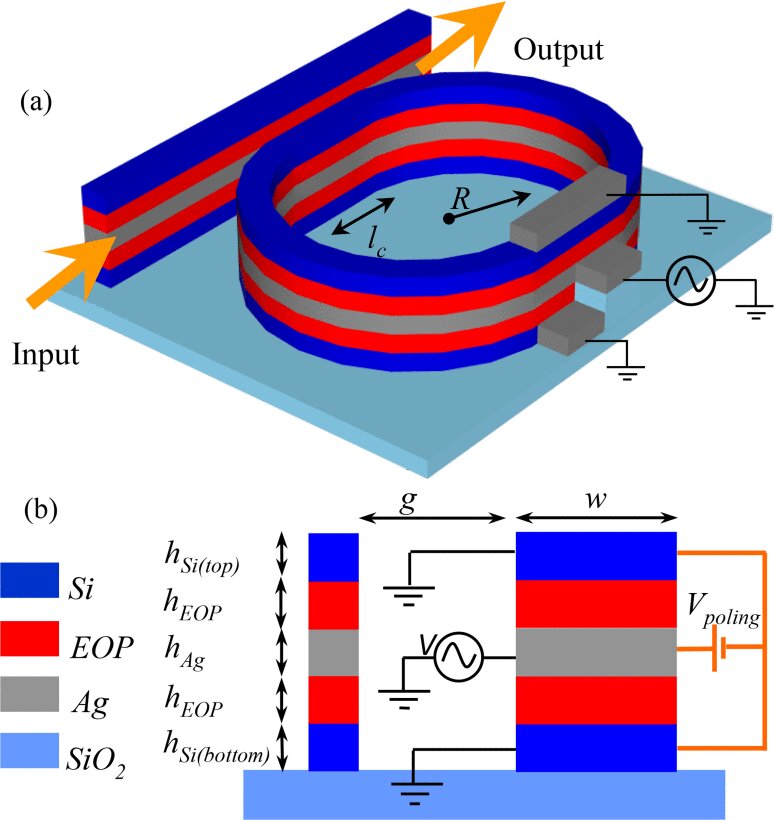
(a) Perspective view of a racetrack resonator coupled to a bus waveguide. (b) Cross-section view showing the gap g between the racetrack and the straight waveguide. Courtesy of IEEE Xplore.
Applications of Microresonators
Microresonators have primarily been investigated for their high wavelength selectivity characteristic. When the optical path length of a roundtrip in the resonator is equal to an integer number of the wavelength, the light constructively interferes or “builds up” inside the ring. The optical path length depends on the design and the intrinsic parameters of the ring such as the radius and the index of refraction. By changing the refractive index of the resonator or varying the radius of the resonator, the resonant wavelength can be tuned to the desired wavelength. This property drove the integration of microresonators in many filtering applications including add-drop filters. However, in order to realize higher order filters and achieve better performance, microresonators are usually cascaded in series or in parallel as shown in the figure below. Sometimes, more complex configurations are required.
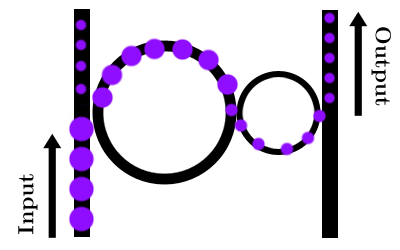
Example of cascaded ring resonators. Courtesy of Wikimedia Commons.
Microresonators are also characterized by their high sensitivity to changes in the effective refractive index making them good candidates for temperature sensing applications. Since the effective index of refraction varies with temperature, very small changes in temperature can be detected by microresonators. These components are also used to measure the birefringence of waveguides thanks to their polarization sensitivity property. By applying force or mechanical strain to the resonator, its geometry changes thus changing the resonant wavelength. This property can be exploited to create a mechanical sensor. Additionally, microresonators were proven to be useful for realizing optical switches, logic gates, modulators, isolators and multiplexers that are all crucial for optical signal processing. This is owed to the microresonator’s small size, low power consumption, operation efficiency, and possibility of large-scale integration in photonic integrated circuits.
Another interesting property of microresonators is their sensitivity to their environment. Recent research advances have shown that the response of the microresonator changes with the type of chemical solution it is injected with. Therefore, it can be used in biosensing applications to identify molecules and even atoms.
Unlike other devices, a microresonator has many resonant peaks that are equally spaced in the frequency domain. Such functionality has the potential of generating frequency combs useful for signal processing applications.
Conclusion
Microresonator research has evolved over the past two decades. However, there is still a growing interest in these devices and continuous attempts to develop more complex configurations and geometries that are more suitable for advanced applications and easier to integrate with existing photonic devices. Unsurprisingly MEMS research and evolution from research labs to industrial application has seen the use of optical microresonators for implementation of safety and stability systems, optical switches, biometric sensors, mechanical filters, micromirror devices, material characterization, gyroscopes, and more. No wonder some pioneering groups in well known schools have been focusing in these area for the last few decades.
This article was brought to you by World Star Tech - leading provider of solutions in sensing, measurement, biomedical, and laser illumination areas