Introduction
Humankind has had its eyes on the microscopic world for centuries. Jonathan Swift illustrated the fantastically tiny world of the Lilliputians in his classic novel, Gulliver’s Travels. Dr. Seuss imagined a miniature but thriving planet linked to a speck of dust in Horton Hears a Who. The back of Old Spice deodorants humorously advertises their products as containing atomic-sized “scent elves” and “stench-fighting robots”. Despite pop culture’s stereotyping of the micro-realm as fantastical, an enduring scientific interest in the micro-realm, especially in nanomedicine, continues to grow today.
Renowned theoretical physicist Richard Feynman pioneered the concepts that inspired the discipline of nanotechnology in the early 1960s. However, this field only became of scientific interest in the 1980s. Researchers and materials scientists strive to push the boundaries of physics and engineering into the micro-scale, nano-scale, and subatomic-scale. One emerging new advancement in nanotechnology is quantum dots, which have already demonstrated a number of industrial applications. Engineers all over the world are researching quantum dot applications for space exploration, light-emitting diode (LED) design, and photodetection devices. While we have previously covered the subject of quantum dots on this site, this article will focus specifically on its clinical applications in the field of nanomedicine and biodiagnostics.
Background
Quantum dots (abbreviated as QDs) are semiconductor nanoparticles that possess unique optical and electronic properties. These distinct characteristics are explained by their extremely small size, which causes these particles to behave differently than larger-scale particles according to the principles of quantum mechanics.
In the present day, quantum dots are built from binary ionic compounds in a core-shell structure, where the main semiconductive center is covered by a shell of compatible material with differing semiconductivity. Some examples of binary compounds that are used to build quantum dots are:
- lead compounds (lead sulfide, lead selenide)
- cadmium compounds (cadmium selenide, cadmium sulfide, cadmium telluride)
- indium compounds (indium arsenide, indium phosphide)
Nanomedical researchers have also built metal-free quantum dots, using elements like silicon and germanium. These are synthesized in a controlled gas-phase process in nonthermal plasma. When in vivo, quantum dots are pre-coated with a stable polymer shell to prevent cadmium poisoning. The stabilizing polymer coat will also ensure that UV irradiation does not cause any of the quantum dot material to seep into the bloodstream or other tissues.
Basics of Nanophotonics in Quantum Dots
Shining UV light on a sample of quantum dots will excite electrons into higher energy levels. Since these nanoparticles are built from semiconductors, the excitation energy is defined as difference in energy between the valence band and the conductance band. To review, the valence band and the conductance band are the two energy levels closest to the Fermi level of a material; this information is crucial to determining a material’s conductivity. When the electron returns back to ground state, the atom emits the resultant energy as a photon of light. Altering the size of quantum dots will also alter their optoelectronic properties. Quantum dots with larger diameters emit longer wavelengths of light. The largest dots emit red and orange light, and the smallest particles emit blue and violet light.
Applications of QDs in Nanomedicine
On this blog, we have already explored the role of quantum dots in cancer therapy and neural imaging. However, there are a number of other clinical applications that remain unexplored with quantum dots.
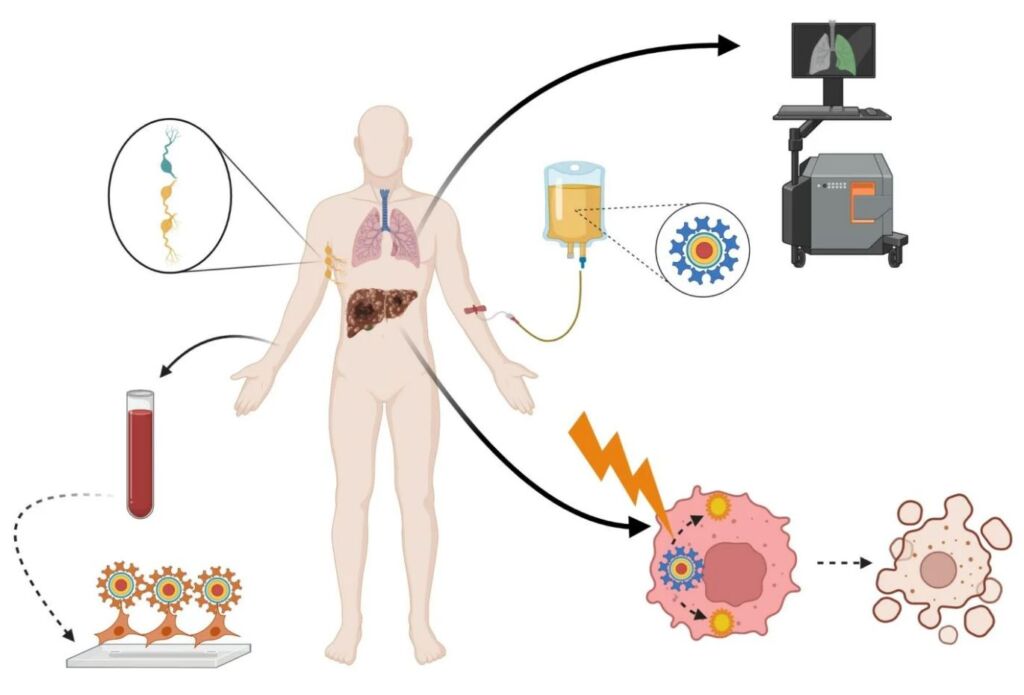
Applications of Quantum Dots in Nanomedicine. Image Courtesy of DovePress
Quantum dot probing has strong potential in becoming a commonplace technique in high-resolution fluorescent imaging. Quantum dot nanocrystals demonstrate extraordinary photostability. As such, scientists can mark bodies of interest with quantum dots, and track their physiological activity for weeks. While the nanomedical research community is still cautious about using quantum dot technology in vivo, scientists are more comfortable with conducting in vitro research projects with this technology, especially in observing single-cell migration in embryonic development and metastatic malignant cancer.
Interestingly, scientists and biomedical researchers have expressed strong interest in studying the antibacterial properties of semiconductive nanoparticles, which includes the study of quantum dots. In Singapore, a research team supported by Nanyang Technological University’s Center for Advanced Bionanosystems discovered that some nanoparticles show promise in fighting bacterial infections on a dose-dependent basis. A separate research team in Iran also published research demonstrating the effectiveness of quantum dots in combating gram-positive and gram-negative bacteria.
Benefits of QDs in Nanomedicine
Quantum dots have proven to be advantageous for fluorescent imaging usage in comparison to traditional organic dyes. First, the brightness levels of quantum dots are much stronger than the usual markers used in fluorescence microscopy. To quantitatively compare the two brightness levels, scientists examine a value called the extinction coefficient, or the attenuation coefficient. The extinction coefficient is a numerical property describing a material’s propensity to reflect certain wavelengths of light. Since quantum dots have a much higher extinction coefficient compared to fluorescent dyes, their resultant brightness is also much stronger.
Second, the stability of quantum dots leads to a reduced photobleaching effect. Photobleaching is the permanent loss of fluorescence from light-induced, irreversible chemical changes to dyes or other fluorophores. The cleaving or denaturing of covalent bonds between the fluorophore and neighboring particles is the chemical basis of the photobleaching effect. Quantum dots have proven that, in comparison to typical organic dyes or fluorescent proteins, they can withstand many more light excitation cycles before becoming completely photobleached.
Drawbacks of QDs in Nanomedicine
Quantum dots also have limitations in their role in biodiagnostics and nanomedicine. As mentioned earlier, UV light may photolytically dissolve quantum dot samples over time. This heightens the risk of toxic components entering the bloodstream or essential tissues. Quantum dot assembly now includes safety measures, so the risk of hazard has greatly reduced in the last decade.
Another reason that quantum dots may prove disadvantageous to other established methods is because of a phenomenon called fluorescence intermittency. Fluorescence intermittency, also known as blinking, is the process of random switching between dark and bright states of the quantum dot under continuous excitation. It turns out this is a common phenomenon at the nanometer scale that is seen in various emitters such as molecular fluorophores, colloidal quantum dots, etc. Unfortunately, this phenomenon is governed by the power-law statistics for the bright and dark time distributions. Because of this any measurements of the time-averaged intensity for the single quantum dot might not be very reproducible.
Final Thoughts
Quantum dots have a lot to offer in terms of potential for industrial and biomedical application. Despite the drawbacks that currently prevent it from clinical usage, quantum dots show a promising future in nanomedicine. As scientists establish further research on the properties of nano-scale semiconductors, it will become evident whether or not quantum dots possess the chemical and fluorescent potential equivalent to its current impression.
Today's blog post is sponsored by Connet Laser Technology - the leaders in the development of fiber laser technologies