Recent advances in neural imaging (or neuroimaging) techniques may pave the way for better treatment of neurological diseases such as Parkinson’s disease and next-generation applications in fields such as computer-brain interfaces. Optical Recording of Intrinsic Signals (ORIS) is a relatively new neuroimaging technique, which allows for high spatial and temporal resolution imaging of brain function using visible light reflected off of the cortex. Scientists at Weill Cornell Medical College have used this powerful technique to perform advanced epilepsy studies in animals and human patients using standard LED, fiber optics, and CCD camera sensors. Their work provides potential for drastic improvement over current clinical neural sensing platforms.
- What is Electroencephalography (EEG)?
- What is Electrocorticography (ECoG)?
- Limitations of Common Clinical Neuroimaging Techniques
- High Resolution Neural Imaging Enabled by Visible Light
- Linking Blood Flow to Epileptic Seizures
- Implantable Devices as an Alternative to ECoG
- Some External Resources on Neuroimaging
1. What is Electroencephalography (EEG)?
One of the most common methods for mapping neural activity on the surface of the brain is to measure changes in the local electrical field potential via an array of metal surface electrodes placed on top of the scalp. This technique is called electroencephalography, or EEG.
When the neurons in the brain fire, there is typically an associated flow of ions within the neuron that results in a fluctuation in the localized electric field. For a single neuron firing within the brain, the change in the local field potential (or LFP) is not significant enough for an EEG array to detect. However, if a large number of neurons are firing in sync, they can cause a large enough change in the LFP for an electrode on top of the scalp to measure. The changes in LFP due to neural activity are recorded for each electrode and usually displayed as a series of what appear to be “squiggles” or “brain waves”.
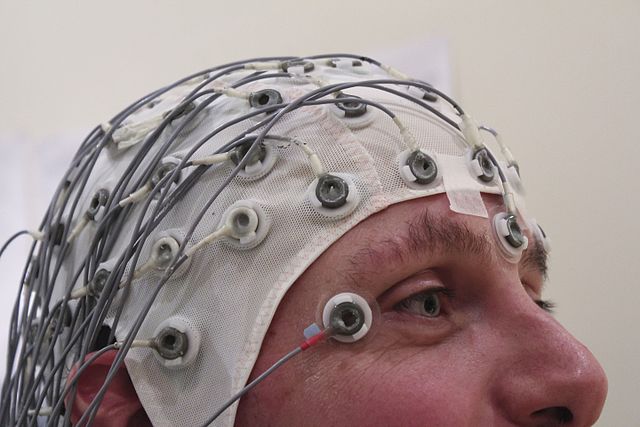
Clinical methods for neural imaging include using EEG surface electrodes to detect changes in the local electric field caused by the firing of neurons. Image by Chris Hope
2. What is Electrocorticography (ECoG)?
EEG arrays is one of the current standard for diagnosing and studying neurological diseases such as epilepsy. However, current clinical hardware itself is highly limited in the spatial resolution of the images since the electrical signals in the brain are scattered due to cerebral brain fluid, bone, and tissue between the cortex and the electrodes. On the other hand, the minimally invasive nature of EEG measurements make them ideal for long term studies of patients where lower spatial resolutions are acceptable. As a result, clinicians often use EEG to get a general idea of localized neural activity in response to conditions such as epilepsy before performing a more invasive or involved study.
For cases where invasive neural surgery may be inevitable, higher fidelity and higher spatial resolution can be achieved using electrocorticography, or ECoG. ECoG uses the same operating principles as EEG, but places the surface electrodes directly on the surface of the cortex. Patients requiring long term ECoG monitoring may undergo a procedure where the top of the skull is surgically removed, the electrode array is implanted on top of the brain tissue, and then finally the skull is replaced on top of the sensor.
3. Limitations of Common Clinical Neuroimaging Techniques
While implanted ECoG sensors are capable of performing continuous measurements in ambulatory patients over a long period of time, current clinical ECoG sensors attain limited imaging resolution of around 1 cm x 1 cm. Surgeons performing resections of the brain aim to completely remove the minimum amount of brain matter that can treat the patient’s symptoms, so an uncertainty on the order of a centimeter can mean any combination of missing the removal of problematic tissue necessitating additional surgery or resecting healthy brain tissue that results in hampered brain function.
While there are a number of alternate methods for producing high spatial resolution maps of a neural activity such as functional magnetic resonance imaging (fMRI), positron emission tomography (PET), and autoradiography, most of these techniques require the patient to be completely stationary and in some cases require the use of potentially harmful dyes. In the case of epilepsy patients preparing for surgery, long-term monitoring of neural activity over the course of days up to a few weeks is necessary to properly identify the target area of resection, ruling these techniques out.
Considering all these, there remains to be a clinical solution for performing highly continuous, long-term neural imaging at high resolutions in ambulatory patients, but new technologies and imaging techniques using optoelectronic sensors may pave the way to an improved alternative to EEG and ECoG arrays.
4. High Resolution Neural Imaging Enabled by Visible Light
The ability to measure brain function using visible light was first demonstrated by scientists over 30 years ago. This technique, often referred to as optical imaging or optical recording of intrinsic signals (ORIS), measures light reflected off of the exposed cortex of an animal. Depending on the wavelength of light used, ORIS can detect specific neural activity and functions in the reflected light. Measurements of light reflectivity do not directly measure the electrical neural activity or brain function the same way an EEG or ECoG array does. Instead, changes in the reflectivity of the tissue occur due to blood flow, electrical activity, or metabolic activity.
Scientists at Cornell Weill Medical College have demonstrated the ability to detect and localize seizure activity in the brain using ORIS techniques in a paper published in 2006. The scientists produced high resolution 2D maps of blood flow in the brain in real time using light reflected off of the cortex which in turn revealed where seizure activity was occurring on the surface of the brain. To achieve this, narrow wavelength LED light was guided into the cortex through optical fiber and the reflected light was captured by a CCD or CMOS camera sensor mounted over the exposed brain. Using this technique, scientists were able to localize blood flow (and indirectly measure neural activity linked to epilepsy) in the brain down to a 100 µm x 100 µm resolution in both rats and human patients.
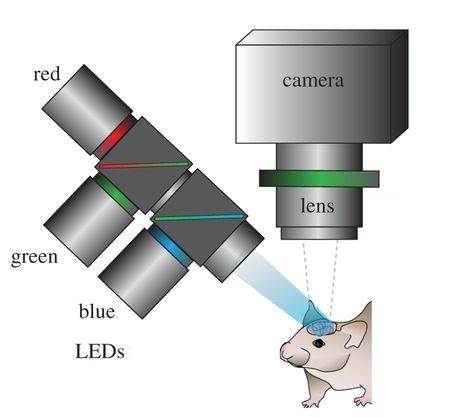
Optical recording of intrinsic signals creates high resolution maps of blood flow in the brain. This allows for the accurate detection of seizures. Image by Ma, et al.
5. Linking Blood Flow to Epileptic Seizures
In the event of a seizure, neural activity in the afflicted region enters an unregulated positive feedback loop, which can consequently trigger the same behavior in adjacent neurons. Focal seizures are classified by seizure activity that originates in a specific region (called the focus) and then spreads to the surrounding tissue. The focus of the seizure is a typical target for surgical resection in severe epilepsy patients. However, the difficulty in identifying the location of the focus with precision is a primary challenge working against the success of surgical treatments for epilepsy.
Neural imaging of epileptic activity using ORIS relies on the behavior of the cerebral blood flow in the brain. Under normal conditions, the body’s vasculature supplies blood to the brain with low frequency, sinusoidal oscillations occurring due to neural activity and certain metabolic responses of the brain tissue. However, during a seizure, the exaggerated neural activity causes the afflicted regions of the brain to inundate with blood, which ORIS measurements record as a sharp dip in reflectivity due to absorption of light by the hemoglobin in blood. ORIS measurements are sensitive to the wavelength of light used for illuminating the tissue. This enables the use of different wavelengths to determine such parameters as blood oxygenation in addition to total blood volume.
6. Implantable Devices as an Alternative to ECoG
Using ORIS, the epileptic focus was located in animals and human patients with a precision on the order of 100 µm. This is over a 1,000x improvement over EEG and ECoG which has a precision on the order of 1 cm. Unfortunately, the animals and patients in these experiments were observed for short periods of time while sedated with their brain surgically exposed. Much like fMRI or autoradiography, standard ORIS measurement setups require a stationary patient. This makes them unsuitable for clinical uses requiring continuous measurement of patients over a long period of time.
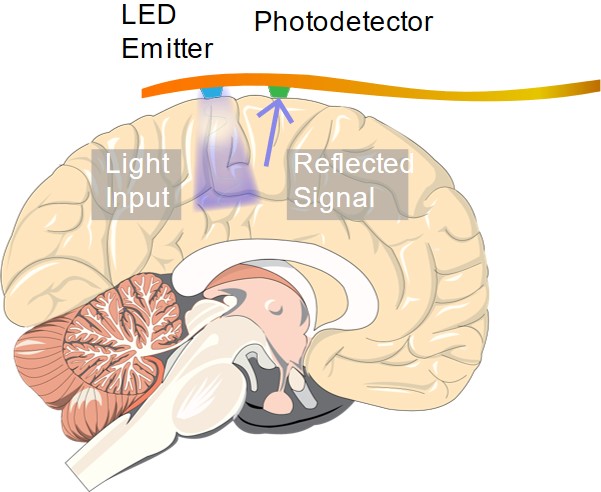
An implantable device capable of ORIS measurements. May enable continuous clinical high-resolution neural imaging measurements over long periods of time
In order for a technique like ORIS to compete with ECoG in diagnosing epilepsy patients and neurosurgery candidates, an implantable sensor capable of performing high resolution optical measurements is necessary. In another article, we discuss research efforts on developing implantable optical sensing technologies that may enable the next generation of medical sensors for neural imaging.
This article was brought to you by World Star Tech - leading provider of solutions in sensing, measurement, biomedical, and laser illumination areas.
7. Some External Resources on Neuroimaging
- How does ORIS detects localized epileptic seizure activity. Molecular Neurobiology volume 33, pages181–197 (2006) External Link.
- EEG cap image by Chris Hope (https://www.flickr.com/photos/tim_uk/8135755109/). Image used under CC 2.0 License
- Schematic image of ORIS setup – altered and taken from: Ma, Ying, et al. “Wide-field optical mapping of neural activity and brain haemodynamics: considerations and novel approaches.” Phil. Trans. R. Soc. B 371.1705 (2016): 20150360. Image used under CC 4.0 License.
- Grinvald, Amiram, et al. “Functional architecture of cortex revealed by optical imaging of intrinsic signals.” Nature, 324.6095 (1986): 361-364.
- Suh, Minah, et al. “Neurovascular coupling and oximetry during epileptic events.” Molecular Neurobiology, 33.3 (2006): 181-197.