Embark on a voyage into the shadowy realm of neutrino oscillations, where elusive particles shift identities and challenge our cosmic understanding. This concise guide lays the groundwork for a deeper dive into the physics phenomenon that garnered Nobel recognition. Explore further in the table of contents.
Table of Contents
- Introduction to Neutrino Oscillations
- The Nature of Neutrinos
- Unraveling the Mystery of Neutrino Oscillations
- Key Experiments and Discoveries
- The Nobel Prize in Physics
- The Implications of Neutrino Oscillations
- Experimental Techniques and Technologies
- Neutrinos in the Wider Universe
- Conclusion
- Additional Resources
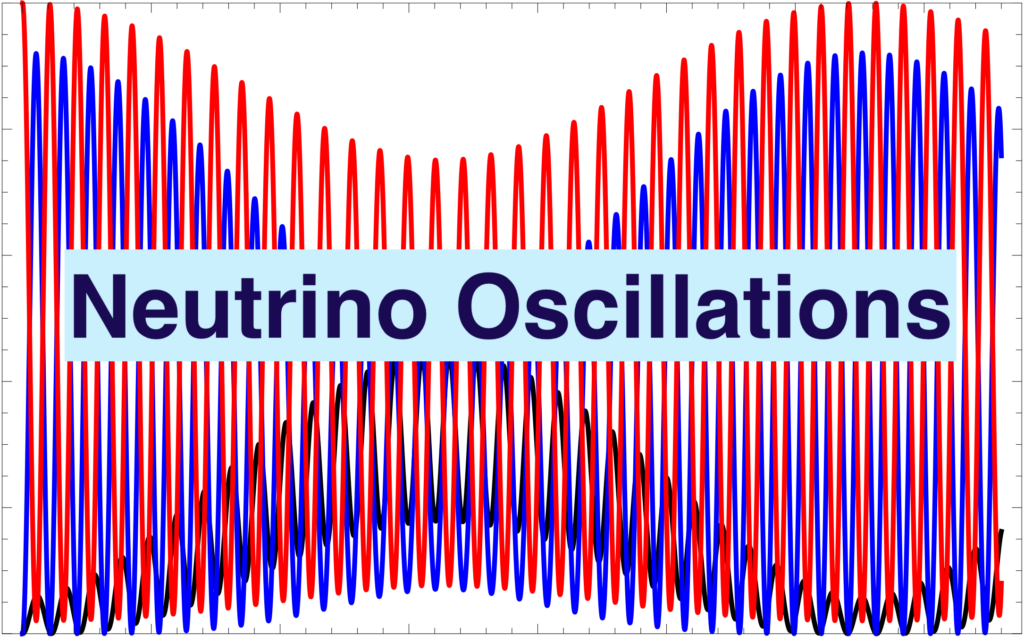
This graph captures the essence of neutrino oscillations, where neutrinos switch identities as they traverse space, a groundbreaking discovery that reshaped our understanding of these elusive particles.
1. Introduction to Neutrino Oscillations
Neutrinos, elementary particles with near-zero mass and no electric charge, are integral to our understanding of the universe’s fundamental forces. These particles, produced in vast quantities by nuclear reactions in stars, including our sun, barely interact with matter, making them extremely difficult to detect and study. The concept of neutrino oscillations, a quantum mechanical phenomenon where neutrinos switch between their three known types (flavors) – electron, muon, and tau – as they travel, challenges core principles of particle physics and suggests that neutrinos, contrary to previous beliefs, possess mass.
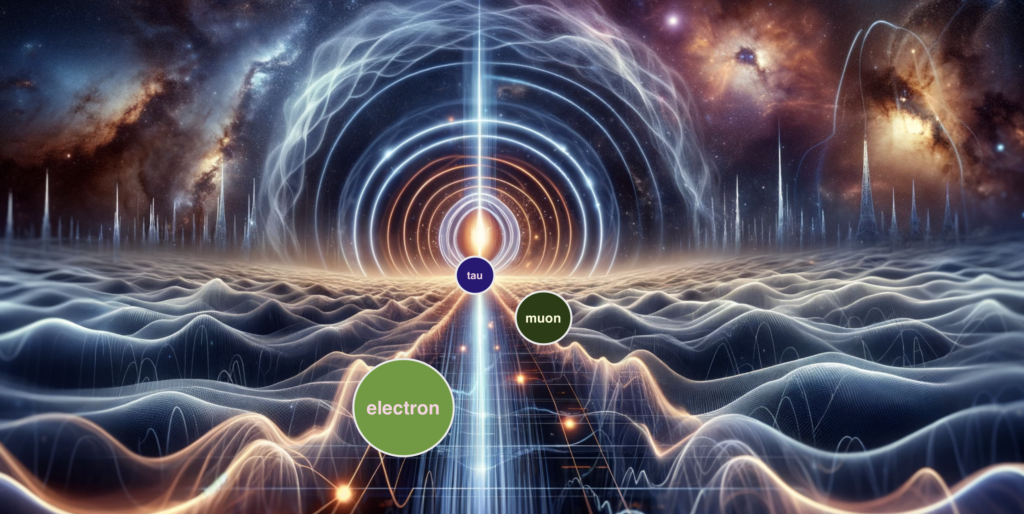
This image illustrates a neutrino as it oscillates between its three identities: electron, muon, and tau, showcasing the fundamental process of neutrino oscillations.
This revelation has profound implications for the Standard Model of particle physics, which initially assumed neutrinos to be massless. The discovery of neutrino oscillations has been a pivotal moment in the field, highlighting the intricate and often surprising nature of subatomic particles. It underscores the importance of neutrinos in understanding the universe’s smallest building blocks and the forces that govern their interactions. The Nobel Prize awarded for this discovery acknowledges its critical role in advancing our comprehension of both the microcosmic particles that make up the universe and the overarching principles that guide their behavior. As research continues, neutrino oscillations remain a key area of inquiry, promising to unlock further mysteries of the cosmos and refine our models of the universe.
2. The Nature of Neutrinos
Neutrinos, elusive subatomic particles integral to the fabric of the universe, were first proposed by Wolfgang Pauli in 1930 to account for missing energy in beta decay processes. However, it wasn’t until 1956 that physicists Clyde Cowan and Frederick Reines confirmed their existence through the Cowan-Reines neutrino experiment, detecting neutrinos produced by a nuclear reactor. This groundbreaking discovery earned Reines the Nobel Prize in Physics in 1995, acknowledging the significance of bringing these ghostly particles to the realm of observed phenomena. The term “neutrino,” coined by Enrico Fermi, originates from the Italian diminutive for ‘little neutral one,’ aptly naming these neutral particles.
Unique Properties and Behaviors:
Neutrinos are remarkably characterized by their minimal interaction with matter and lack of electric charge. Their ability to pass through entire planets without any interaction makes them incredibly challenging to detect. Neutrinos exist in three flavors: electron, muon, and tau neutrinos, corresponding to their lepton counterparts. Intriguingly, they can oscillate between these flavors, a quantum phenomenon indicating that neutrinos have mass, albeit extremely small.
These particles are omnipresent, with trillions passing through our bodies every second, originating from the sun, cosmic events, and human-made sources like nuclear reactors. A majority of the neutrinos floating around were born around 15 billions years ago, soon after the birth of the universe. Since this time, the universe has continuously expanded and cooled, and neutrinos have just kept on going. Theoretically, there are now so many neutrinos that they constitute a cosmic background radiation whose temperature is 1.9 degree Kelvin (-271.2 degree Celsius).
Role in the Standard Model of Particle Physics:
Within the Standard Model of particle physics, neutrinos are essential components, categorized under leptons. Despite their elusive nature, neutrinos play a crucial role in maintaining the balance of forces in the universe, participating in weak interactions—one of the fundamental forces governing particle interactions. The discovery of neutrino mass and oscillations posed a challenge to the Standard Model, prompting revisions to accommodate these particles’ mass properties. Understanding neutrinos and their behavior not only enriches the Standard Model but also offers insights into the early universe’s conditions and the mechanisms driving cosmic events.
3. Unraveling the Mystery of Neutrino Oscillations
Unraveling the mystery of neutrino oscillations has been a pivotal journey in the realm of particle physics, marked by theoretical predictions, perplexing problems, and groundbreaking experimental evidence.
Theoretical Prediction of Neutrino Oscillations
The concept of neutrino oscillations first emerged from the mathematical framework laid out by Bruno Pontecorvo in the late 1950s. He proposed that neutrinos, much like the neutral kaons in quantum mechanics, could oscillate between different types, a phenomenon that would imply these particles have mass. This idea challenged the prevailing assumption of massless neutrinos in the Standard Model and suggested a deeper complexity within the quantum world.
The Solar Neutrino Problem
The solar neutrino problem surfaced in the 1960s when Raymond Davis Jr.’s pioneering experiment detected significantly fewer electron neutrinos from the sun than theoretical models predicted. This discrepancy pointed towards a fundamental gap in our understanding of neutrinos and their interactions. The solution to this problem lay in the realization that neutrinos could change flavors from the electron type, which the experiment was designed to detect, to muon or tau types, which would go undetected in Davis’s detector, effectively ‘disappearing’ and leading to the observed deficit.
Experimental Evidence Supporting Neutrino Oscillations
The definitive proof of neutrino oscillations came from a series of experiments in the late 1990s and early 2000s. The Super-Kamiokande experiment in Japan observed the transformation of muon neutrinos into tau neutrinos, while the Sudbury Neutrino Observatory (SNO) in Canada provided clear evidence that solar neutrinos were oscillating between different types. These findings not only solved the solar neutrino problem but also confirmed the mass-bearing nature of neutrinos, revolutionizing our understanding of these enigmatic particles and their role in the universe.
These developments underscored the dynamic and ever-evolving nature of particle physics, illustrating how theoretical predictions, coupled with innovative experimental techniques, can unravel some of the most profound mysteries of the cosmos.
4. Key Experiments and Discoveries
The journey to understanding neutrino oscillations is marked by several key experiments that have significantly contributed to the detection and understanding of this phenomenon. These experiments not only confirmed the existence of neutrino oscillations but also provided invaluable data that deepened our understanding of neutrinos and their behavior.
The Homestake Experiment: The Homestake Experiment, conducted in the late 1960s in a gold mine in South Dakota, was one of the first to observe the discrepancy in the number of neutrinos detected from the sun, compared to theoretical predictions. Led by Raymond Davis Jr., this experiment used a large tank filled with perchloroethylene, a cleaning fluid, to detect solar neutrinos. The results, showing fewer neutrinos than expected, hinted at the possibility of neutrino oscillations, contributing to the “solar neutrino problem.”
Kamiokande and Super-Kamiokande: The Kamiokande (Kamioka Nucleon Decay Experiment) and its successor, Super-Kamiokande, located in Japan, played pivotal roles in observing neutrino oscillations. Super-Kamiokande, in particular, with its vast tank of pure water surrounded by sensitive detectors, provided clear evidence of neutrinos changing types. The experiments led by Masatoshi Koshiba, and later efforts at Super-Kamiokande under Takaaki Kajita, were instrumental in confirming the phenomenon of neutrino oscillations through atmospheric neutrino observations.
Sudbury Neutrino Observatory (SNO): The Sudbury Neutrino Observatory in Canada, led by Arthur B. McDonald, further advanced the understanding of neutrino oscillations by detecting solar neutrinos through heavy water. SNO was able to observe all three types of neutrinos, providing direct evidence of neutrino oscillation and resolving the solar neutrino problem by confirming that electron neutrinos from the sun were indeed transforming into muon and tau neutrinos.
Contributions of Key Scientists
The contributions of key scientists such as Arthur B. McDonald and Takaaki Kajita have been monumental in the field of neutrino physics. McDonald’s leadership in the SNO experiment and Kajita’s work with the Super-Kamiokande experiment were critical in providing the experimental proof for neutrino oscillations, a discovery that earned them the Nobel Prize in Physics in 2015. Their work not only confirmed the theory of neutrino oscillations but also opened new avenues for research in particle physics, cosmology, and the understanding of the fundamental forces that govern the universe.
5. The Nobel Prize in Physics
The 2015 Nobel Prize in Physics was awarded to two pioneering scientists, Takaaki Kajita from Japan and Arthur B. McDonald from Canada, for their significant contributions to the discovery of neutrino oscillations—a phenomenon indicating that neutrinos change identities. For several decades physicists tried to solve a perplexing mystery about neutrinos. A set of calculations had predicted exactly how many neutrinos must be created in the nuclear reactions that power the sun. But when measurements were done on earth, it appeared that two thirds of the neutrinos were missing. Where had the neutrinos gone?
The Discovery of Neutrino Oscillations
McDonald’s and Kajita’s discovery showed that the neutrinos didn’t disappear at all but merely changed identities. In late 90’s Dr. Kajita’s experiments suggested that neutrinos created upon the interaction of cosmic rays with the earth’s atmosphere did not truly disappear but switched between two identities. Neutrinos were once thought to be massless, but decades of study have led them to conclude that their collective weight in the cosmos is about equal to the collective weight of stars.
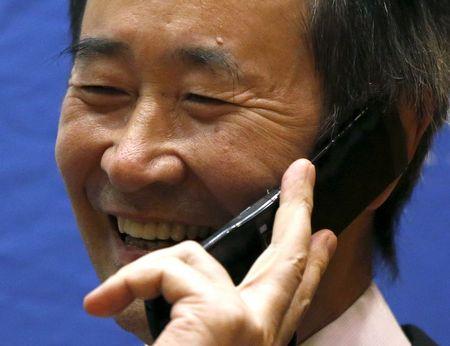
Takaaki Kajita, director of the Institute for Cosmic Ray Research and professor at University of Tokyo, smiles as he speaks with Japanese Prime Minister Shinzo Abe at the start of a news conference in Tokyo October 6, 2015. REUTERS/Issei Kato
Kajita, leading the Super-Kamiokande detector in Japan, and McDonald, directing the Sudbury Neutrino Observatory in Canada, provided key experimental evidence that neutrinos oscillate, which implies that these subatomic particles have mass. Their groundbreaking work not only solved the long-standing solar neutrino problem but also opened new horizons in understanding the universe’s fundamental constituents.
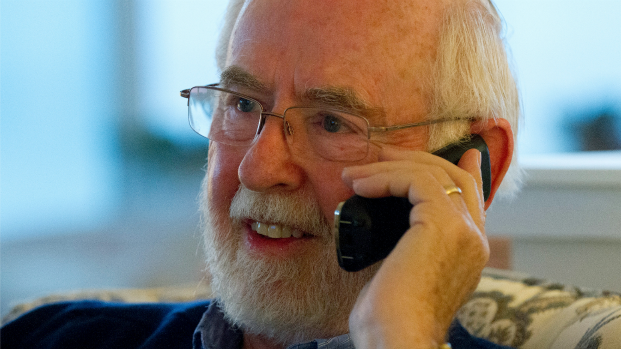
Professor Arthur McDonald, Arthur “Art” Bruce McDonald, is a Canadian astrophysicist and the Director of Sudbury Neutrino Observatory Institute. He also holds Gordon and Patricia Gray Chair in Particle Astrophysics at Queen’s University in Kingston, Ontario. You can read more about him on his Wikipedia Page
The Nobel Prize acknowledgment of neutrino oscillations highlighted their critical role in particle physics and cosmology, driving increased research, collaboration, and investment. This recognition not only celebrated the groundbreaking contributions of McDonald and Kajita but also reinforced the collective endeavor of the scientific community to decipher the universe’s fundamental mysteries through advanced experiments and studies.
6. The Implications of Neutrino Oscillations
The discovery of neutrino oscillations has profound implications for the field of physics, challenging and expanding our understanding of the fundamental constituents of the universe.
Neutrino Mass and Its Implications for Physics:
The phenomenon of neutrino oscillations directly implies that neutrinos have mass, a revelation that necessitates revisions to the Standard Model of particle physics, which originally accounted for massless neutrinos. This discovery has prompted physicists to reconsider the nature of mass itself and explore beyond the current model to accommodate these elusive particles. The nonzero mass of neutrinos adds a new dimension to our understanding of the universe’s smallest building blocks, influencing theories about the fundamental forces that govern particle interactions.
Role in Understanding the Universe:
Neutrino oscillations offer a unique window into processes occurring at the most fundamental level in the cosmos. As neutrinos interact only weakly with matter, they can traverse the universe virtually unimpeded, carrying information from the most distant and ancient astrophysical events. This makes them invaluable probes for studying cosmic phenomena, such as supernovae and the behavior of matter under extreme conditions. Furthermore, the fact that neutrinos have mass contributes to the total mass-energy balance of the universe, affecting models of cosmic evolution and the large-scale structure of the cosmos. Their role in dark matter research is particularly intriguing, as neutrinos present a viable candidate for the elusive dark matter that exerts gravitational influences yet eludes direct detection.
Current and Future Research Directions:
Understanding neutrino oscillations has opened new avenues of research and inspired a global effort to study these particles in greater detail. Current research focuses on pinpointing the exact masses of the three neutrino types, investigating the possibility of additional neutrino types (sterile neutrinos), and exploring the role of neutrinos in the early universe. Future experiments aim to utilize neutrinos in novel ways, such as using neutrino detectors to monitor nuclear reactors for non-proliferation efforts or probing the Earth’s interior. The ongoing study of neutrino oscillations continues to push the boundaries of our knowledge, promising to uncover further mysteries of the quantum world and the vast universe beyond.
7. Experimental Techniques and Technologies
Detecting and studying neutrinos, given their elusive nature, requires innovative technologies and methods. To capture these ghostly particles, scientists have developed specialized detectors placed in deep underground, underwater, or ice environments to shield them from cosmic rays and other background noise.
Detection Methods: The primary technique for neutrino detection involves large volumes of water or heavy water, where neutrinos interact with atomic nuclei or electrons, producing detectable light signals in a process known as Cherenkov radiation. Detectors like Super-Kamiokande in Japan utilize thousands of tons of pure water surrounded by sensitive photomultiplier tubes to catch these faint light signals, allowing the observation of neutrino interactions.
Innovations in Neutrino Observatories: Innovations in neutrino observatories have expanded the reach and capabilities of neutrino research. Underwater observatories, such as ANTARES in the Mediterranean Sea, and ice-based detectors like IceCube at the South Pole, leverage their massive volumes of natural water and ice to create vast detection areas, enabling the capture of high-energy neutrinos from cosmic sources. These observatories not only push the limits of neutrino detection but also contribute to our understanding of cosmic events and the fundamental properties of neutrinos.
These advanced technologies and methods are pivotal in the ongoing quest to unravel the mysteries of neutrinos and their oscillations, providing insights into the fundamental laws of the universe and opening new frontiers in particle physics and cosmology.
8. Neutrinos in the Wider Universe
Neutrinos play a pivotal role in cosmic events and contribute significantly to our understanding of the universe’s composition and behavior.
Cosmic Events and Supernovae: In cosmic cataclysms like supernovae, the explosive death of stars, neutrinos are abundantly produced, carrying away a vast amount of energy. These neutrino bursts provide critical information about the mechanics of supernova explosions and the nuclear processes at their cores, offering insights into the life cycles of stars and the synthesis of heavy elements in the universe.
Cosmic Radiation Background: Neutrinos also form a part of the cosmic radiation background, a relic from the early universe. Just seconds after the Big Bang, neutrinos decoupled from other matter, freely streaming through space ever since. Studying this neutrino background can reveal crucial details about the universe’s infancy and its subsequent evolution, complementing our understanding derived from the cosmic microwave background radiation.
Future Missions and Experiments
Looking ahead, a range of future missions and experiments are poised to delve deeper into the neutrino enigma. Projects like DUNE (Deep Underground Neutrino Experiment) in the United States and Hyper-Kamiokande in Japan aim to study neutrino oscillations with unprecedented precision, investigate the possibility of CP violation in the neutrino sector, and explore the role of neutrinos in the early universe. These endeavors, along with initiatives to detect the cosmic neutrino background directly, promise to open new windows onto the universe’s most fundamental processes and structures.
Through these multifaceted roles and future explorations, neutrinos stand at the forefront of our quest to understand the vast cosmos, from the most explosive events to the silent, expansive stretches of the universe.
9. Conclusion
Neutrino oscillations have emerged as a cornerstone of modern physics, challenging and enriching our understanding of the universe’s fundamental constituents. This phenomenon not only revealed that neutrinos have mass, overturning long-standing assumptions in the Standard Model of particle physics, but also highlighted the intricate dance of particles that underpins the fabric of the cosmos.
The journey to unravel the mysteries of neutrinos reflects the broader quest of physics to decipher the universe’s most fundamental elements and forces. Each discovery in the realm of neutrino physics has opened new avenues of inquiry, pushing the boundaries of what we know about the cosmos, from the smallest scales of particle interactions to the vastness of cosmic evolution.
As we stand on the cusp of new discoveries, the field of particle physics continues to beckon researchers, students, and science enthusiasts to explore its depths. The study of neutrinos, with its blend of mystery, challenge, and potential, represents a vibrant and dynamic frontier in our quest to understand the universe. The encouragement for further exploration and study in this field is not just an academic pursuit; it is a call to adventure in the vast, uncharted territories of knowledge that await the curious and the bold.
prof premraj pushpakaran writes — 2018 marks the 100th birth year of Frederick Reines!!!
Professor Prem raj Pushpakaran writes — 2022 marks the birth centenary year of Leon M. Lederman!!!