Researchers at the University of Colorado, Boulder have been experimenting with laser sources called optical frequency combs to find a new way of studying molecular structures. Frequency combs get their name from the fact that the spectra of these lasers are divided into uniformly spaced lines. It turns out that these lasers can become an ideal tool for molecular spectroscopy if optically tuned to mid-infrared frequencies. As we will see later, their peculiar properties give these lasers distinct advantages over other light sources.
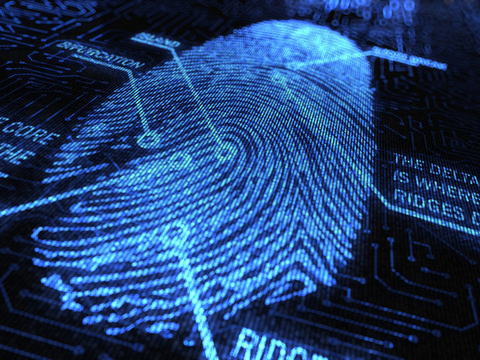
Photo retrieved from CPOA, at Flickr.
In a work, conducted by the UC Boulder scientists, they describe their method of generating a very broadband optical frequency combs. With a simple fabrication, these researchers were able to harness the infrared tale of the soft x-ray light output. In doing so, they achieved the brightness and spectral resolution that enabled them to carry out high-precision, dual-frequency-comb (DFC) spectroscopy on the vapors of methanol and ethanol.
What is Molecular Fingerprinting?
Understanding and categorizing molecular compositions is a major thrust in modern chemistry and material science. Hence, understanding and identifying molecular sub-structures, that gives us hints of similarity between molecules are paramount for making advances in this field. With the development of spectroscopy, using light sources to identify molecular contents has proven to be a powerful way of studying such structures.
In essence, molecular fingerprinting is a way to encode a molecule’s structure for easy reference of its contents. To get the specifics on a molecule’s design, researchers often carry out spectroscopy that involves light in the infrared region of the optical spectrum: 6.7 to 20 micrometers. Studying molecules in this spectral range often provides essential information about their chemical structures and the makeup. Over time, this gave rise to a term coined by researchers as “fingerprinting”.
IR spectroscopy is an important tool in molecular fingerprinting. By studying the interaction of light with the vibrational states of the molecule, we can learn a lot about their vibrational energy states, which in turn hint us about their physical structure and identify unique characteristics. Because often this vibrational states lie in the IR part of a spectrum and occupy a rather wide domain, they impose rather specific conditions on the parameters of light. In particular, this means that the light used should have a broadband spectrum capable of exciting various molecular energy states within a molecular structure.
What Tools are Available for Molecular Spectroscopy?
Fourier transform infrared spectroscopy (FTIR) has been one of the standard techniques to produce broadband radiation capable of identifying molecular characteristics. It uses black body radiator type light sources that produce polychromatic IR radiation. A form of absorption spectroscopy, FTIR, uses the mathematical process of Fourier Transformation to convert range of measurements into spectral information.
In a standard FTIR spectrometer, the IR beam, containing many frequencies shines through an interferometer onto a sample and measures how much of the beam is absorbed by the sample. By repeating the measurement with different sets of frequencies, researchers obtain a series of data points that they feed into a computer program for analysis. Subsequently, a computer converts the light’s distance/time series data, into frequency spectra using a Fourier transformation program.
Pros and Cons of Various Light Sources
Blackbody radiation type light sources are convenient, relatively affordable and compact enough to fit into commercial table-top FTIR spectrometers. However, these light sources have some limitations, primarily driven by the low range of propagation, lack of focus and spectral sensitivity. Moreover, the accuracy of the beam is affected by divergence that occurs in the interferometer, which can further reduce the spectral resolution.
More recently, scientists turned to massive facilities like the Large Hadron Collider, for high-quality light outputs to study different molecules in a variety of settings. These operations typically contain synchrotron light sources, which can create bright, infrared light for larger scale studies than FTIR. Synchrotron light sources are usually capable of producing soft x-rays as a byproduct of accelerating electrons at an angle perpendicular to their velocity. Moreover, because of their high intensity and broadband spectrum, their IR tail becomes rather suitable for molecular studies.
While such synchrotron facilities generate high quality beams, the complexity, size and the cost often makes them prohibitive and inaccessible for many laboratories to carry out practical experiments. With this in mind any table-top systems capable of producing similar quality light sources become very essential. One such advance in this direction is the work done by the UC Boulder researchers, who designed a rather compact table-top light source capable of producing soft x-rays with output beam characteristics at par with those generated with giant synchrotron facilities.
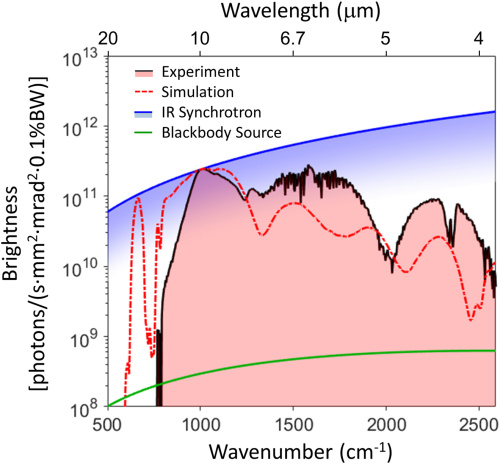
The figure above compares the brightness output of different light sources. The researcher’s simulation greatly outpaces black body lamps in terms of brightness, and they reach the same peak brightness as infrared synchrotron sources. Accessed via OSA Publishing.
How do Optical Frequency Combs Improve Molecular Fingerprinting?
Optical frequency combs have precise frequencies, with a larger bandwidth and better data processing methods than FTIR, which makes them a clear alternative to polychromatic light sources. As mentioned in the introduction, the researchers use DFC to prevent any gaps in resolution. This is the use of two optical frequency combs, whose repetition rates stagger to fill in the gaps that each comb leaves. This allows the two combs to compensate for any losses in resolution that the other may have.
Difference frequency generation (DFG) takes place to generate the two combs of the spectra. Employing DFG, the researchers were able to obtain optical frequency combs with mid-infrared wavelengths that allowed them to perform spectroscopy on gases – vapors of methanol and ethanol. The dual comb setup, ensures wide spectral coverage while maintaining a relatively high resolution.
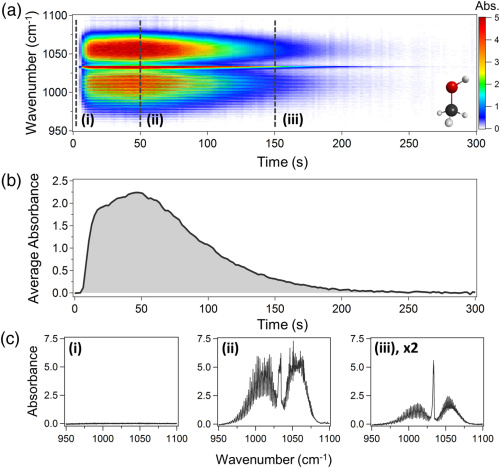
The image above is a time series display of methanol evaporation as collected by the researchers using infrared frequency combs. Figure A shows the DCS spectra as the methanol evaporates. Figure B is a graph of the beam’s average absorbance, showing that it detects changes in methanol composition. C is another graph that shows the beam’s exact absorbance at three points in the spectra from A. Accessed via OSA Publishing.
Applications of Optical Frequency Combs
This creative use of DFG in combination with optical frequency combs has a potential in a wide range of molecular study applications. The setup simplifies the creation of broadband, infrared light capable of advanced spectroscopic studies without relying on large synchrotron facilities. This opens up avenues and accessibility to use these tools in many laboratories for expanded studies of various chemical compounds and even biological species. The dual frequency comb method can further be advanced by engineering these setups into compact integrated photonics form factors that might just commercialize these versatile tools.