Optogenetics is the emerging field of using light to control and study neuronal activity. In 2005, a Stanford University team was able to use a light-sensitive microbial protein to control the timing of neuron pulse firing. Since then many protein compounds have been used to control neuronal activity and study centers of the brain. Especially previous hard to study areas such as the amygdala. In 2010, Nature Methods named optogenetics the “Method of the Year” across various disciplines in science and engineering. It is a cross field discipline which demands in-depth command of knowledge in chemistry, biology, physics, and engineering to bring about meaningful advances.
Apart from the uses of imaging and control of neurons to a previously unprecedented precision, optogenetics offers promise for treating neuronal diseases. By being able to inhibit or increase neuronal activity, scientists are seeking to treat such ailments as Parkinson’s disease, epilepsy, and even depression.
In this article, we will discuss the principles of optogenetic actuators and sensors; the common proteins used; and the various lasers that are used for these purposes. We will also discuss some potential applications towards the end of this post.
Mechanism of Controlling Neuron Activity
Neurons communicate to each other through action potentials and synapses. The cell membrane looks like a capacitor which carries a constant voltage. At a synapse, a neuron has many ion gated channels that only let the ions flow into the neuron if certain neurotransmitters are present. These ions then trigger a severe change in the membrane potential referred to as an action potential. The action potential then travels down a neuron.
In optogenetics, light sensitive proteins are used to induce or inhibit action potentials. No neurotransmitters are necessary for this purpose. The protein molecules are special in that they open certain ion channels when hit with a particular wavelength of light. This then triggers an action potential. The opposite effect of inhibiting action potentials can also be achieved at a different wavelength. Thus by modulating the wavelength, one can control neuronal activity.
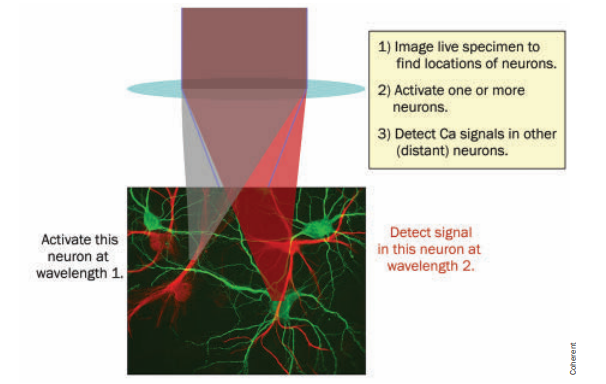
Different wavelengths used for neuronal actuation and imaging. Courtesy PhotonicsSpectra.
Why has this not been tried before? Apart from finding the right proteins for this purpose, LEDs do not offer good spatial and temporal resolution as the beam profile is bigger. Surface emitting lasers solve this problem because of the beam profile beaming smaller in spot size, which can focus on individual cells. 3D locatization in neuronal control can be achieved along with sensors designed for 3D imaging. Currently research is trying to increase the number of neurons controlled and imaged simultaneously.
The mechanism of light-protein gated channels is illustrated in the below image.
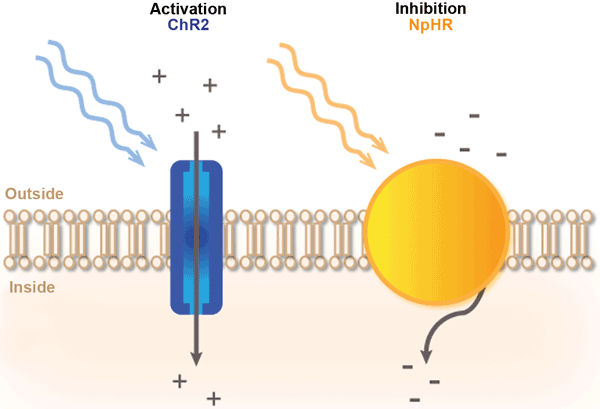
Blue light triggers channelrhodopsin, and yellow light triggers halorhodopsin. Both have opposing effects. Courtesy PhotonicsMedia.
Protein channelrhodopsin (ChR2) is used to activate the movement of Calcium ions into the neuron with blue light. For the opposing effect, halorhodopsin (NpHR) is used to close the ion gated channels with yellow light.
Proteins Used in Optogenetics
The key to optogenetics is finding the right light-sensitive proteins. Channelrhodopsin, halorhodopsin, and archaerhodopsin are the commonly used proteins. Channelrhodopsin and its variants are modulated around 473 nm. Halorhodopsin is activated around 660 nm, and archaerhodopin lies in the middle around 561 nm. Since these frequencies are mostly in the visible range, typical diode lasers operating in this wavelength range can be used. This is not an exhaustive list as higher wavelength compounds and lasers have also been used.
There are two systems for an optogenetic setup: optogenetic actuators, and optogenetic sensors. Optogenetic actuators involve using lasers to activate or inhibit the light-sensitive protein molecules as detailed above.
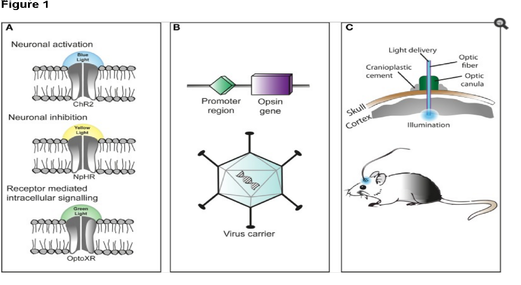
Left: Light-controlled protein ion channels. Yellow and blue light are used for modulation. Right: Setup for experimentation on a rat’s brain. Courtesy Wiki.
Using High-Energy Femtosecond Lasers
Femtosecond lasers can be used as better actuators. This is because they can trigger multi-photon generation for better control of neuronal activity. Moreover, the effect is more localized as non-linear effects take place only at the beam waist. It also exerts minimal photo-damage because of its localization. Multi-photon use also enables using lasers in the infrared region of 1040-1150 nm. Ytterbium doped fibers with optical parametric oscillators come as a powerful tool here as they are tunable in the the desired range to excite and inhibit and offer higher energies needed for multi-photon absorption.
Sensors for measuring neuronal activity are designed by measuring the concentration of calcium or neurotransmitters. A wavelength used for imaging is different from the wavelength used for sensing. The total setup for actuation and imaging is as shown in the below image.
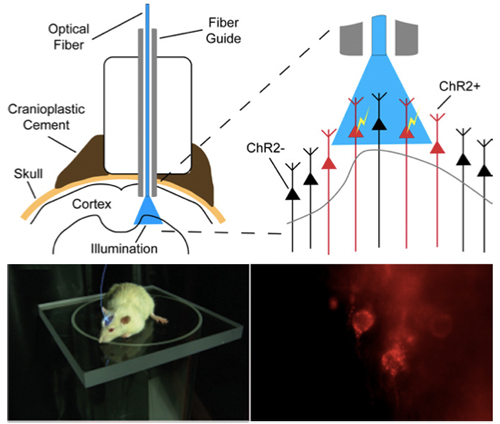
Setup of an optogenetic system to study and control neuronal activity in a mouse. Light is fiber guided to the region of application.
Future Applications
Optogenetics has been proposed as a potential method to treat Parkinson’s disease and epilepsy. For example, there are special molecules designed to glow red with high influx of Ca ions. These ions can trigger the nearby optogenetic cells to inhibit their neuronal function. A series of such reactions can help prevent intense brain activity such as during epilepsy. Optogenetics has also been proposed for other cells in the other parts of the body. For example, Ca ions have an important role in insulin production. If high concentrations of Ca ions are designed to glow red, they can excite the cells involved in insulin production optogenetically.
As we saw in this post, optogenetics is one more area of frontier science that heavily relies on diode lasers, or as demonstrated in a more recent research work, femtosecond lasers to push the envelopes of science and technology.
If you liked this article, you might also like our post on DNA Sequencing through Photonics.