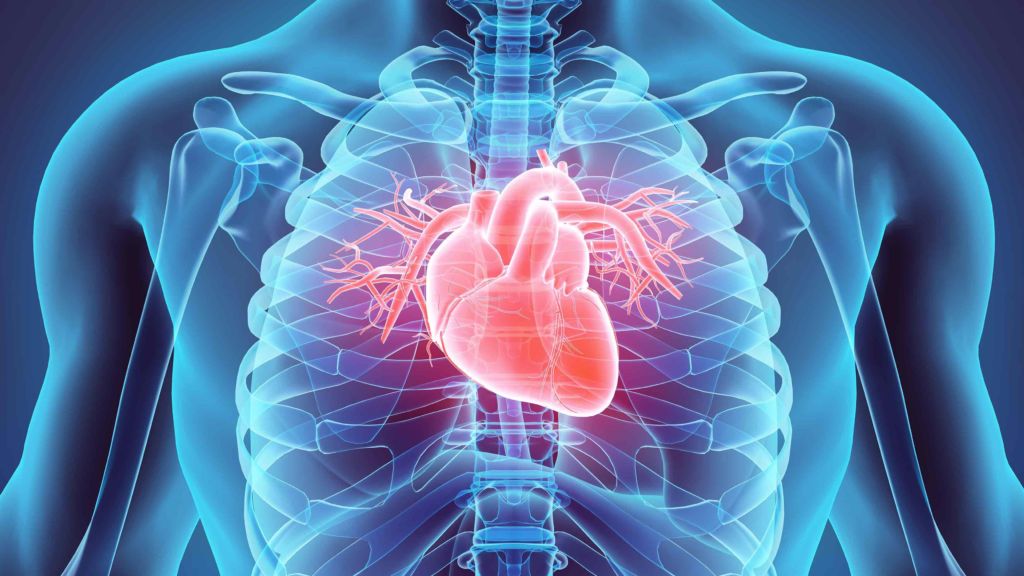
Photoacoustic imaging provides a viable method for early diagnosis of many cardiovascular diseases. Courtesy of National Heart, Lung, and Blood Institute.
Cardiovascular Disease and Photoacoustic Imaging
Cardiovascular diseases (CVDs) are the number one cause of death in the world, accounting for 31% of all deaths. This class of diseases includes heart failure, angina, and many others that involve the heart or blood vessels. Most CVDs are associated with atherosclerosis, the buildup of plaque within the arteries. Atherosclerosis can quickly become fatal when the plaque suddenly ruptures and triggers blood clots, for serious clotting often causes heart attacks and strokes depending on the site of the artery. Atherosclerosis, however, is a slowly progressive disease. When detected early, patients are able to take medication and make appropriate lifestyle changes to dramatically slow down or even reverse the buildup.
Recent studies of human aortic plaque suggest rupture is more likely where there is a high concentration of lipids. This could be an extremely useful indicator for prevention purposes, but current imaging techniques will require additional modification for accurate identification. A collaborative research team with South China Normal University and Shandong University recently proposed a new photoacoustic endoscope for lipid-rich plaque imaging in last month’s Optics Express issue. This novel method promises high-resolution intravascular images for identifying vulnerable atherosclerosis plaque.
Introduction to Photoacoustic Imaging
Optical coherence tomography and ultrasound are currently the two main intravascular imaging techniques. These methods have proven to be extremely effective in certain biomedical applications, but they both produce limited results for lipid detection. The team chose to use photoacoustic imaging to obtain high-resolution images at significant penetration depths. Photoacoustic imaging is a relatively new technique that combines the high-contrast of optical imaging with the high spatial resolution of ultrasound imaging.
Photoacoustic imaging delivers a pulsed laser into the body where it is absorbed by biological tissue. This causes the tissue to undergo thermoelastic expansion and emit a characteristic photoacoustic signal. The magnitude of this signal is proportional to the local energy deposition. Photoacoustic imaging can therefore identify chemical components with significant sensitivity. The research team uses this technique to identify plaque components and map lipid concentration.
Method and Design
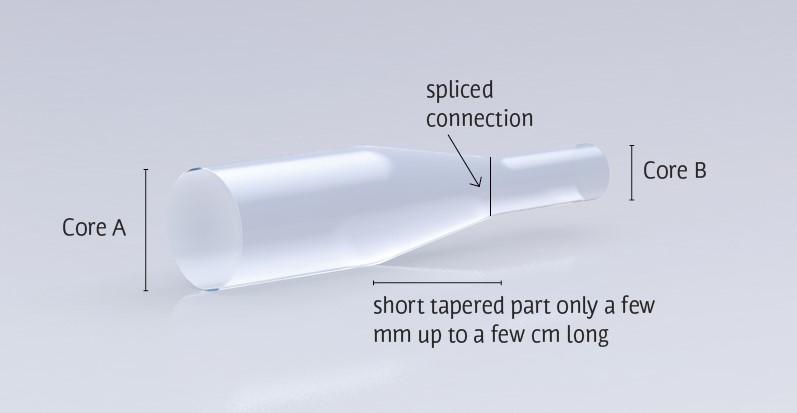
Tapered fibers allow a collimated beam to gain significant energy density. Courtesy of CeramOptec.
The Tapered Fiber
The novelty of this device is in the fiber choice. The research team chose a Ø25-Ø9 tapered fiber whereas most photoacoustic biomedical experiments employ a single-mode fiber. This system must be able to deliver light with high enough energy to overcome the effects of attenuation in water. Otherwise, insufficient laser energy will not excite photoacoustic signals from the lipids. Single-mode fibers provide high resolution, but they cannot carry high optical power due to low damage threshold at the air/fiber interface.
To overcome this challenge, many proposed photoacoustic systems have utilized a multimode fiber due to their high coupling efficiency and damage threshold. These fibers, however, provide poor lateral resolution and excitation efficiency because of imaging system space constraints. By using a tapered fiber the research team creates an imaging system capable of delivering high energy laser output without sacrificing resolution.
The Complete Setup
The intravascular photoacoustic (IVPA) imaging system uses a DPSS pulsed laser source. An achromatic objective lens reshapes and focuses the pulsed beam into the 25 micron core diameter end of the tapered fiber. The light then transmits from the 9 micron core end of the fiber into a spliced single-mode fiber of the same core diameter and finally into the endoscopic probe.
This probe contains a C-lens and 55° reflector to focus the beam on the object of interest. An ultrasound transducer detects the emitted photoacoustic signal which travels via a coaxial line to a rotary joint at the end of the single-mode fiber. The rotary and torsion coil pullback device are the main scanning mechanisms. Finally, a field-programmable gate array (FPGA) triggers the laser, pulse generator, and a data-acquisition card. The card digitizes and records the signal to a computer.
The Results
The research team conducted a series of tests to demonstrate the imaging abilities of their intravascular imaging system. The design of their probe allows the researchers to directly compare image results from photoacoustic and ultrasonic signals.
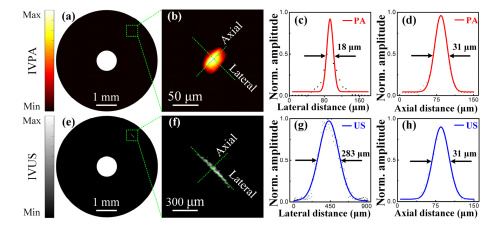
Data shows significantly improved resolution when comparing photoacoustic (PA, top row) to ultrasound imaging (US, bottom row). Courtesy of Optics Express
Lateral and Axial Resolution
The team first imaged a 7 micron carbon fiber to confirm the lateral and axial resolution of the photoacoustic/ultrasonic system. They found the IVPA system achieved a lateral resolution of 18 microns and axial resolution of 31 microns; meanwhile, intravascular ultrasound (IVUS) imaging provided lateral resolution of 283 micron and axial resolution of 31 microns.
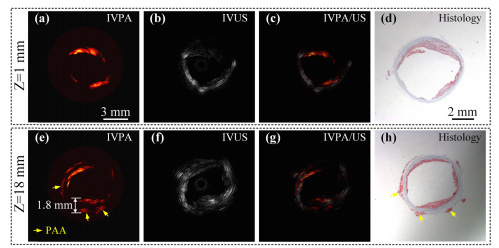
IVPA, IVUS, IVPA/US, and histological images (from left to right) at positions Z=1mm and Z=18mm. Courtesy of Optics Express.
Lipid Distribution and Concentration
The researchers then acquired 3D images of a lipid-rich rabbit aorta that was set in water to mimic the intravascular environment. They compared IVPA and IVUS images to histological sections of the aorta to determine how accurately their imaging system could identify lipid distribution. At a shallow imaging depth (Z=1mm) lipid distribution determined by IVPA closely matches that of the histological sections. The research team was also able to confirm exceptional imaging capabilities at Z=18mm. At this position, IVPA imaging showed lipids in the inner wall of the aorta as well as periadventitial adipose tissue. This was once again matched with the stained histological slices. This experiment provides powerful evidence that the IVPA system is capable of deep-penetration and high-resolution imaging.
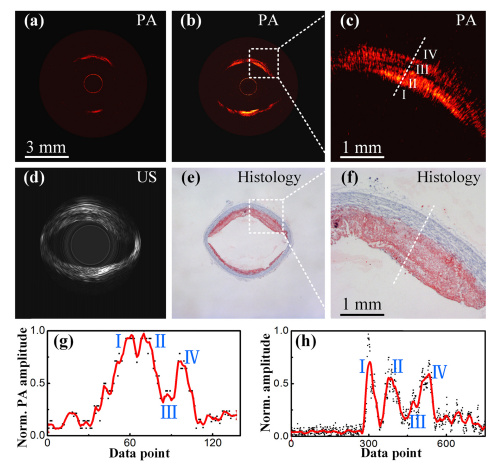
A comparison of the single-mode fiber (a) and tapered fiber (b) with expanded lipid regions (c), IVUS (d), and histological sections (e-f). Figures (g) and (h) portray the correlation between IVPA signal and lipid concentration. Courtesy of Optics Express.
Comparing Fiber Performance
Finally, the research team compared the imaging capabilities of endoscopes with a single-mode fiber with those of a system with the tapered fiber. For this experiment they placed the rabbit aorta in a blood environment. The data clearly shows that the tapered fiber system provides a much more accurate depiction of lipid distribution than the single-mode fiber. The team also notes that the IVPA image depicts multiple layers of lipids that are confirmed by the histological section. They plotted curves for the IVPA data and analyte concentration across these regions (I-IV) as represented by the dashed line in Figure (c). The plots show positive correlation between the amplitude of the photoacoustic signal and relative concentrations. This indicates that the tapered fiber IVPA imaging system successfully detected higher lipid concentrations in regions I, II, and IV.
Concluding Thoughts
The research team has proven that using a tapered fiber for intravascular endoscopy could greatly improve cardiovascular disease diagnosis. This method provides greater laser output energy for deep-penetration and high-resolution imaging. Experiments show the tapered fiber IVPA imaging system can accurately identify lipid distribution and concentration in both water and blood environments. The data indicates that this is not only an effective method in early diagnosis of atherosclerotic plaque, but also a fast and accurate indicator of plaque that could be susceptible to rupturing.
For more information about this potentially life-saving device, find the research team’s article here. If you liked this article you might also like our review of colonoscopy alternatives.