Photonic crystals enable precise control of light at the nanoscale. Their ability to manipulate light through engineered periodic structures has made them an important tool in applications like optical filtering, LEDs, and laser technology. In this article, we examine the principles behind photonic crystals and their impact on photonics, with a focus on practical applications and current advancements.
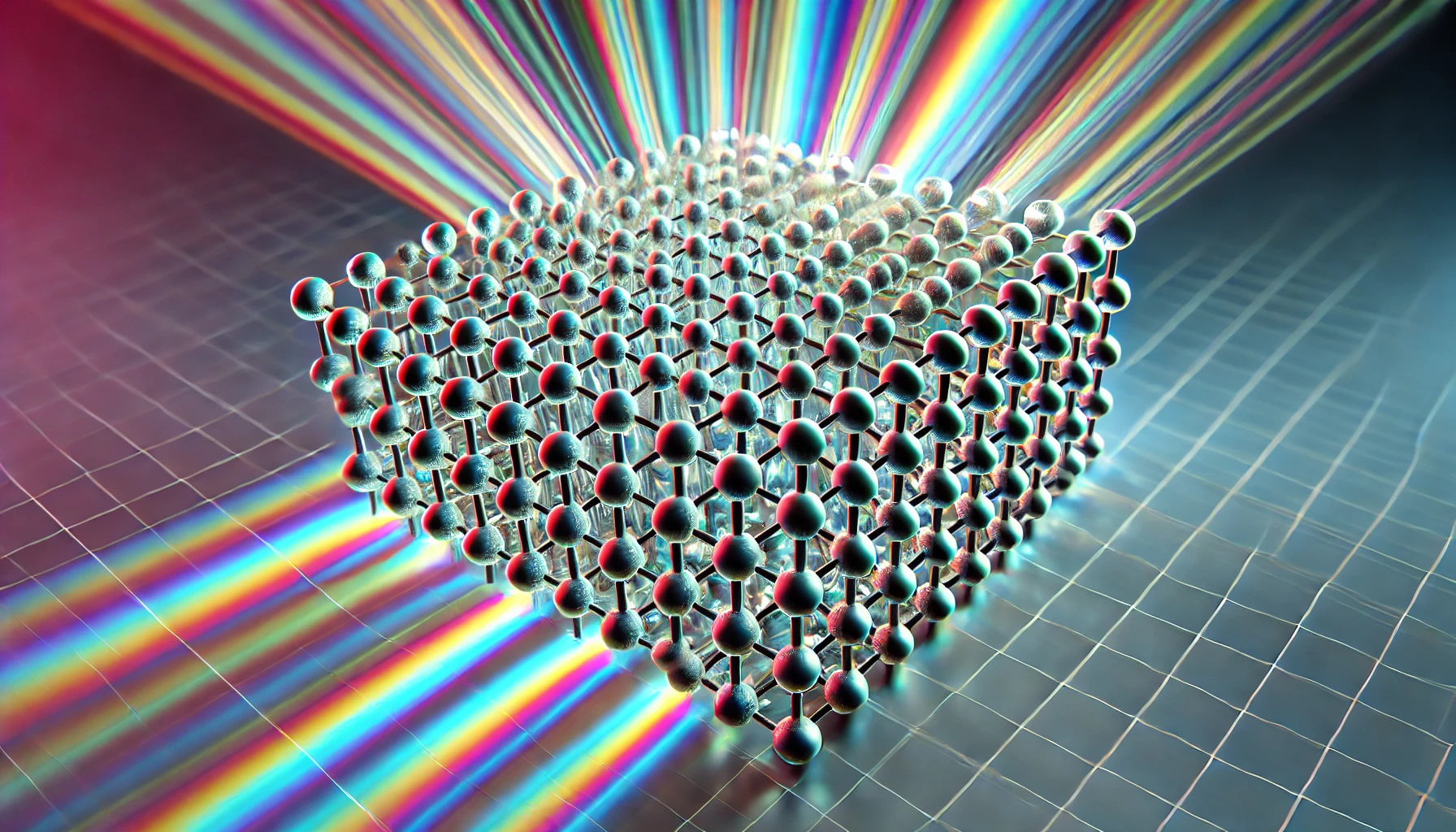
Photonic Crystals: Photonic crystals are engineered structures that precisely control light at the nanoscale by leveraging periodic patterns to create photonic bandgaps. These innovations enable advancements in optical filtering, LEDs, lasers, and more, revolutionizing modern photonics with applications across industries.
This article is sponsored by Gentec-EO – Manufacturer of laser power & energy meters, as well as terahertz meters and beam profiling cameras. Check out their Laser Power Meter Kit for precise and reliable laser measurement solutions tailored to your photonics needs.
1. Introduction
Photonic crystals are engineered nanostructures that have transformed our ability to manipulate light. First proposed in the 1980s, these structures are inspired by the natural periodic patterns found in materials like opals and butterfly wings, which selectively reflect certain wavelengths of light. By creating artificial periodic structures, scientists have harnessed the ability to control light propagation in unprecedented ways.
Photonic crystals have evolved significantly since Lord Rayleigh first studied one-dimensional periodic dielectric stacks, such as Bragg mirrors, in 1887. These early studies identified photonic bandgaps, enabling precise control of light reflection and transmission. In 1987, Eli Yablonovitch and Sajeev John published groundbreaking papers that formalized the concept of photonic crystals, focusing on three-dimensional structures and their impact on spontaneous emission and light localization. Advances in fabrication techniques, such as lithography and self-assembly, have since enabled the creation of two- and three-dimensional photonic crystals. Today, researchers use these structures in diverse applications, including photonic crystal fibers, LEDs, and integrated photonic chips.
In modern photonics, photonic crystals play a critical role in technologies that require precise light manipulation. They are integral to optical filtering in telecommunications, enhancing efficiency in LEDs, and enabling highly efficient laser systems. Their versatility has made them indispensable across various industries, from communications to healthcare and renewable energy.
In this article, we explore the principles behind photonic crystals, breaking down their operation and highlighting their practical applications. By the end, readers will gain a clear understanding of their significance and how they continue to shape the field of photonics.
2. What Are Photonic Crystals?
Photonic crystals are periodic optical nanostructures designed to control the propagation of light. These structures are characterized by a repeating pattern of materials with varying refractive indices, which creates photonic bandgaps — ranges of wavelengths where light cannot propagate through the crystal. This phenomenon enables precise manipulation of light, including reflection, guiding, and confinement.
A useful analogy can be drawn between photonic crystals and semiconductors. In semiconductors, the periodic atomic structure creates electronic bandgaps, which govern the movement of electrons and define the material’s electrical properties. Similarly, photonic bandgaps restrict the movement of photons, determining the optical behavior of photonic crystals. This parallel highlights their foundational role in both optical and electronic systems.
Photonic crystals are categorized into three main types based on their dimensionality:
- 1D Photonic Crystals: These are simplest, consisting of alternating layers of materials with different refractive indices, such as Bragg gratings.
- 2D Photonic Crystals: These are planar structures with periodic variations in two dimensions, often used in photonic crystal fibers and integrated photonics.
- 3D Photonic Crystals: These are the most complex, with periodicity in all three dimensions. They are challenging to fabricate but offer greater control over light, including full photonic bandgaps.
A scanning electron microscope (SEM) image showcasing a two-dimensional photonic crystal made from self-assembled polymethyl methacrylate (PMMA). This intricate nanostructure demonstrates the periodic arrangement of PMMA spheres, enabling the manipulation of light through photonic bandgap effects. Image courtesy of Wikipedia.
Their precise control over light propagation makes photonic crystals fundamental to technologies such as optical filters, waveguides, and light sources.
3. How Do Photonic Crystals Work?
Photonic crystals control light by creating photonic bandgaps — specific ranges of wavelengths that cannot propagate through the structure. These bandgaps result from the periodic arrangement of materials with differing refractive indices. When light interacts with this periodicity, certain wavelengths undergo Bragg diffraction, where constructive and destructive interference patterns emerge. This phenomenon determines whether light is transmitted, reflected, or confined.
Several parameters influence the behavior of photonic crystals. The lattice constant, which defines the spacing of the periodic structure, determines the wavelengths affected by the photonic bandgap. The refractive index contrast between the materials in the periodic arrangement also plays a critical role; higher contrasts lead to more pronounced bandgaps. Additionally, the geometry of the structure, such as its dimensionality and symmetry, directly affects its optical properties.
These principles enable a wide range of behaviors. For example, photonic crystals can guide light along specific paths, even around sharp bends, by confining it within certain regions of the structure. This waveguiding ability has significant applications in photonic circuits. Additionally, photonic crystals can localize light, trapping it in small cavities. This property is crucial for creating efficient laser resonators and optical sensors. By tailoring these parameters, engineers can design photonic crystals to meet specific optical requirements.
4. Applications in Modern Photonics
Photonic crystals have become integral to modern photonics, offering unparalleled control over light propagation. Their unique properties enable diverse applications, from precise wavelength filtering in telecommunications to enhancing the efficiency of LEDs and improving laser performance. In this section, we explore how photonic crystals are applied in optical filtering, LEDs, and laser technologies, highlighting their practical impact and technological significance.
4.1 Optical Filtering
Photonic crystals serve as precise wavelength-selective filters, enabling fine control over the transmission and reflection of light. Their periodic structures create photonic bandgaps that allow specific wavelengths to pass while blocking others. This property is especially valuable in telecommunications, where photonic crystals are used in wavelength division multiplexing (WDM) systems. By isolating individual wavelength channels, photonic crystal filters increase data transmission efficiency in fiber-optic networks.
One notable example is photonic crystal fibers (PCFs). Unlike traditional optical fibers, PCFs incorporate a periodic lattice of air holes running along their length, which influences how light propagates. These fibers excel at filtering and guiding specific wavelengths, making them essential in spectroscopy, sensing, and high-power laser systems.
4.2 LEDs
Photonic crystals improve the efficiency of light-emitting diodes (LEDs) by enhancing light extraction. Without photonic crystals, a significant portion of light generated within the LED remains trapped due to total internal reflection. By incorporating photonic crystal patterns on the surface of LEDs, engineers can redirect trapped light, increasing brightness and energy efficiency.
Photonic crystal LEDs are widely used in high-performance displays, such as in televisions and smartphones, as well as in energy-efficient lighting solutions. The enhanced light output and reduced energy consumption make them critical for sustainable lighting technologies.
4.3 Laser Technology
Photonic crystals have revolutionized laser design by enabling precise control over light emission. By confining and guiding light within photonic bandgap structures, photonic crystal lasers achieve higher efficiency and lower lasing thresholds. These properties allow for compact, low-power, and highly tunable lasers.
A key example is vertical-cavity surface-emitting lasers (VCSELs). These lasers utilize photonic crystal structures to achieve superior beam quality and wavelength stability. VCSELs are widely used in applications ranging from optical communication systems to 3D sensing technologies, such as in LiDAR and facial recognition.
In each of these applications, photonic crystals provide a combination of precision, efficiency, and adaptability, driving advancements across photonics technologies.
5. Current Challenges and Research Trends
Fabricating photonic crystals, especially in three dimensions, remains a significant challenge. Achieving the precision required to construct periodic structures at the nanoscale involves advanced techniques such as lithography, self-assembly, or direct laser writing. These methods often incur high costs, limiting large-scale production and widespread adoption.
Material selection presents another hurdle. To create effective photonic bandgaps, engineers require materials with a high refractive index contrast. However, finding suitable materials that are also durable, transparent, and compatible with existing fabrication processes poses ongoing difficulties.
Research focuses on integrating photonic crystals with emerging technologies. Combining photonic crystals with quantum photonics aims to develop robust platforms for quantum computing and communication. Similarly, incorporating photonic crystals into silicon photonics seeks to enhance performance in optical circuits for data processing and telecommunications. These advancements promise to expand the capabilities of photonic crystals while addressing their current limitations.
6. Conclusion
Photonic crystals have emerged as a powerful tool for controlling light with precision, enabling breakthroughs in optical filtering, LEDs, and laser technologies. Their ability to manipulate light through engineered periodic structures has transformed photonics, driving advancements across industries such as telecommunications, sensing, and renewable energy.
Looking ahead, ongoing innovation in design and fabrication techniques will address current challenges, such as 3D structure production and material limitations. As researchers integrate photonic crystals with quantum and silicon photonics, these structures will unlock new possibilities, solidifying their role as a cornerstone of modern optics and photonic technology.