Introduction
Piezoelectricity is a material’s ability to acquire electric charge in response to an applied mechanical stressor. The root “piezo–” is derived from the Greek word piezein, meaning “to squeeze” or “to press”. Physicist Pierre Curie was the first to discover the piezoelectric effect in 1880. However, industrial manufacturers did not take advantage of this effect until many decades later. Piezoelectric materials have also demonstrated remarkable efficiency when converting mechanical to electrical energy. This has garnered a reputation for piezoelectricity as ensuring exceptional sensitivity and reliability.
Piezoelectric crystals and related materials have been researched as mechanical energy harvesters in search of less polluting, more renewable energy sources. Further technological advancements in microscale and nanoscale materials have led to the conception of miniature piezoelectric generators, with strong potential for adoption by the electronics industry. The medical industry has also found use for piezoelectric crystals in optical ultrasound technology. Biodiagnostic researchers have also been exploring the applications of piezoelectricity over the past five years by developing more efficient, non-traditional molecular affinity assays. The development of piezoelectric immunosensing assays have shown great promise, especially with regards to nucleic acid detection and microbial biosensing.
Background
Piezoelectric crystals can vibrate under the influence of a mechanical stressor or an electric field. The frequency of this vibration depends on the thickness and cut of the crystal. Each piezoelectric crystal has a specific baseline resonant frequency, which changes as mechanical stress causes molecules to adsorb or desorb from the crystalline surface. As such, piezoelectric biosensors are highly sensitive to very small changes in mass.
For any piezoelectric crystal, the change in frequency is proportional to the mass of adsorbed material, up to about a 2% change. There exists a formula to describe the relationship between frequency and mass–
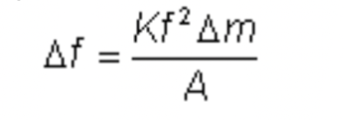
The piezoelectricity formula describing the relationship between frequency and mass.
– where ΔF is the change in resonant frequency (Hz), Δm is the change in mass of adsorbed material, K is a specific constant (dependent on crystal density and cut), and A is the material’s adsorbing surface area (cm2). The increase in mass usually results from oxidation or film deposition at the surface area level.
The electrochemical basis of the piezoelectric effect has much to do with the electric dipole moments in solid materials. In crystals with molecular lattice structures, electrical charges in the surrounding environment induce charge polarization. Because dipoles are vectors, the overall polarization is a vector field. Under the influence of external mechanical stressors, the polarization field will also change. As the solid undergoes reconfiguration, the directionality of the electric dipoles will also change. Sometimes, piezoelectric crystals will experience the opposite effect, called the converse piezoelectric effect. In this phenomenon, when piezoelectric materials are exposed to an electric field of considerable strength, the material may experience mechanical deformation.
Piezoelectric Activity of Quartz Crystals
The most abundant and well-known piezoelectric crystal is quartz. A quartz crystal microbalance (QCM) is a piezoelectrically sensitive apparatus that can determine a materials’ mass variation by detecting the change in oscillation of a quartz resonator. QCMs are, for this reason, have very high efficacy for determining affinity when the material’s surface area is functionalized with recognition sites. With QCMs, researchers can examine affinity behavior for smaller molecules such as simple proteins, as well as for larger polymers and even viruses. QCMs are most frequently used in experiments under vacuum conditions or in the gaseous phase.
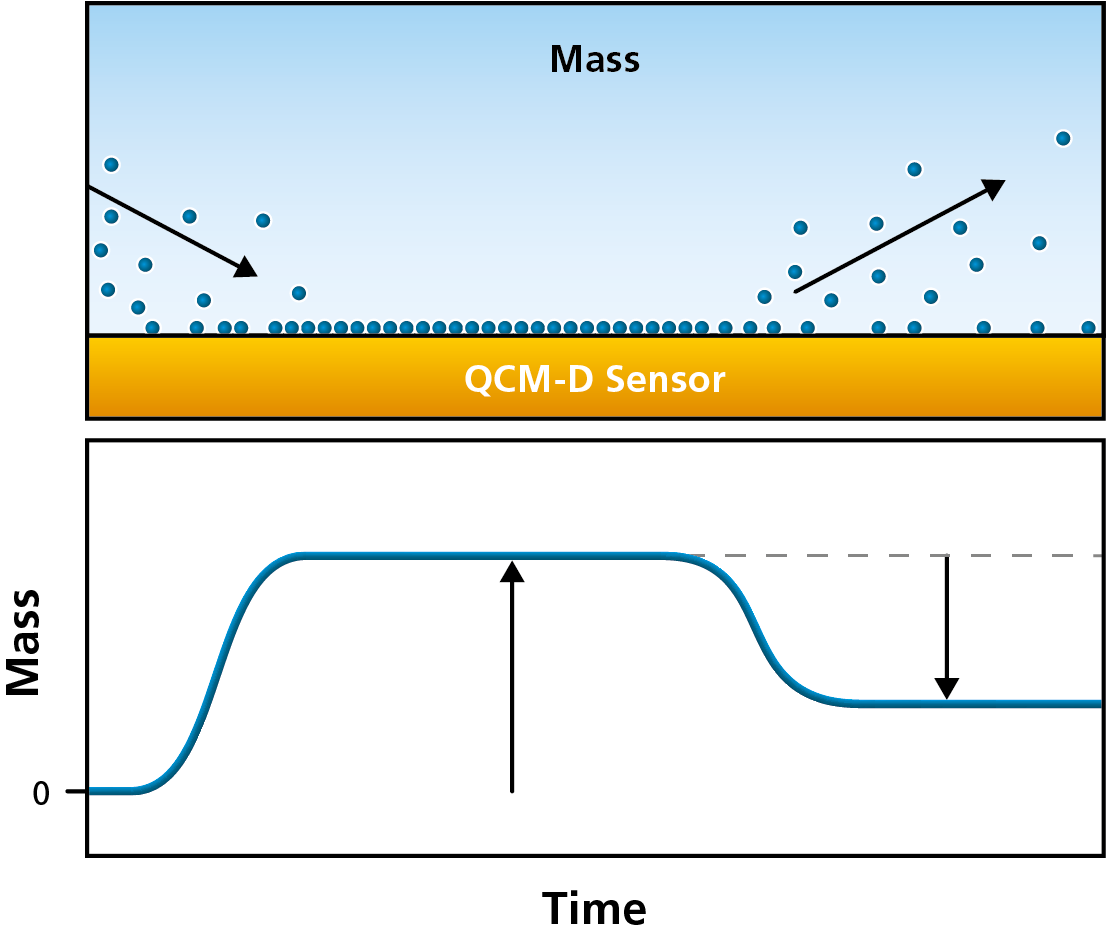
Piezoelectric quartz crystal microbalance sensors can capture minute changes in mass as a function of time. Courtesy of Biolin Scientific.
One simple use of a piezoelectric quartz transducer in biosensors is a formaldehyde biosensor. This utilizes a formaldehyde dehydrogenase coating immobilized to a quartz crystal, which is sensitive to gaseous formaldehyde. Formaldehyde biosensors are inexpensive, small and robust, and can give rapid response times. However, atmospheric humidity may interfere with their readings. It is also difficult to use them to determine formaldehyde concentration in solution.
Piezoelectric Generators
Materials that exhibit piezoelectric activity have also demonstrated potential strength in the renewable and sustainable energy sectors. Piezoelectric materials are capable of harvesting mechanical energy and transducing it into electricity. There are numerous ways to use these materials in the physiological and biomedical industries. Microscale piezoelectric generators could prove useful as a longer-lasting alternative to batteries in biomedical devices. Wearable biomedical device companies have demonstrated interest in adopting this more efficient energy source in their products. This would be particularly beneficial for use in surgically implanted devices, where replacing the battery would be an invasive procedure. Surgeries subject patients to an increased physiological burden, heightening risk of infection as well as other complications. The patients of families may also suffer an undue financial burden with hospital bills and outstanding fees. Undoubtedly, it would serve everyone better to conduct fewer invasive procedures for healthcare maintenance.
Piezoelectric generators may also harvest ambient energy available in vivo. Many physiological processes can generate mechanical energy, especially in the tension force of a heartbeat or lung expansion. Collagen and elastin are abundantly found piezoelectric materials in the body. It should go without saying that these materials thus possess extremely high biocompatibility. Research suggests that skeletal collagen may function as a physiological sensor for force and stress. Soft tissue collagen also exhibits piezoelectric behavior in the Achilles tendon and cardiac walls. By introducing a piezoelectric generator with high biocompatibility into a patient’s body, patients will reap the rewards of increased battery life for some implantation devices, such as pacemakers or hearing aids that rely heavily on electrical energy feedback.
Summary
Piezoelectric materials, specifically crystals, have proven useful across a number of scientific disciplines and manufacturing sectors. These materials possess high moduli of elasticity and great resilience against exposure to powerful electromagnetic fields or intense radiation. These qualities confer a large inherent advantage for industrial application. Combined with a heightened sensitivity and reliability of mass-frequency readouts, piezoelectric materials may also prove to have great potential in the biomedical and biodiagnostic fields. As further research proceeds into the development of quartz-based biosensors and other immunosensing assays, we may approach an era of renewable, sustainable, and longer-lasting energy. Perhaps one day, the majority of our machinery and electronics, from the heavy-duty to microscale, will take advantage of the piezoelectric effect.
This article was brought to you by Zolix Instruments – supplier of scientific instruments and analytical solutions for material science and other applications