Table of Contents
- Introduction to Polarization Control Applications
- Fundamental Concepts in Polarization Control
- Waveplates
- Multiple Order Waveplates
- Zero Order Plates
- Achromatic Plates
- Fiber Optic Polarizers
- Polarization-Maintaining Fiber
- Optical Isolators
- Interferometers
- Laser Resonators
- Advanced Applications
- Future Trends and Research Directions in Polarization Control
- Conclusion and Outlook
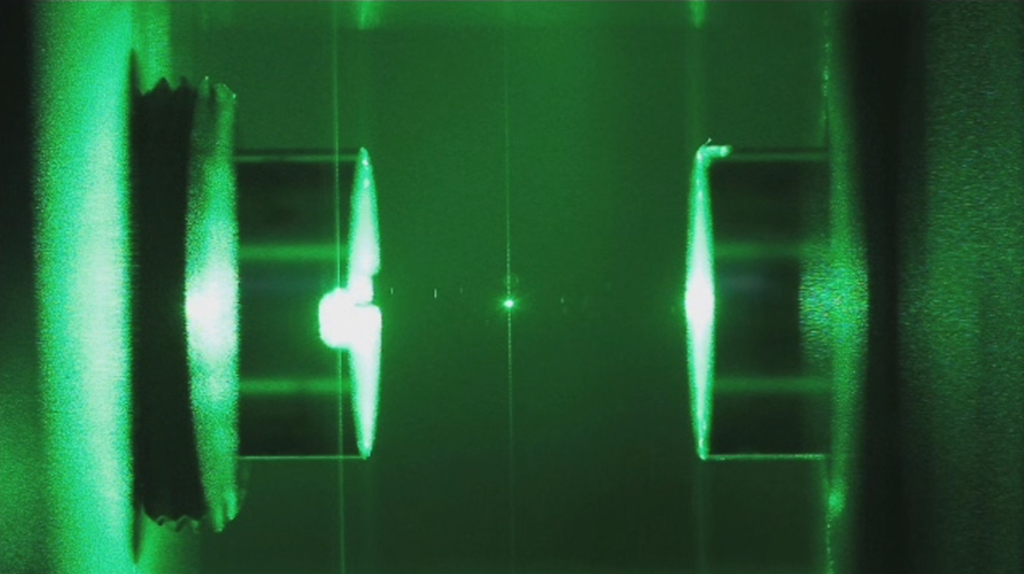
Polarization control devices work to optimize optical performance in many types of systems. Courtesy of Wikipedia.
1. Introduction to Polarization Control Applications
This article builds upon a foundational understanding of polarization and its control mechanisms, delving deeper into the practical applications of polarization control within optical systems. Polarization, a fundamental property of light, significantly influences the efficiency and quality of light transmission in various optical devices and setups. Among the key tools for managing this property are waveplates, fiber optic polarizers, and optical isolators, each serving distinct functions in manipulating light’s polarization to suit specific experimental and industrial needs. These devices are not merely accessories but critical components that enhance the precision and performance of optical systems across a wide range of scientific and technological fields. By examining their application, we aim to shed light on the integral role of polarization control in advancing optical research and its implementation in real-world technologies.
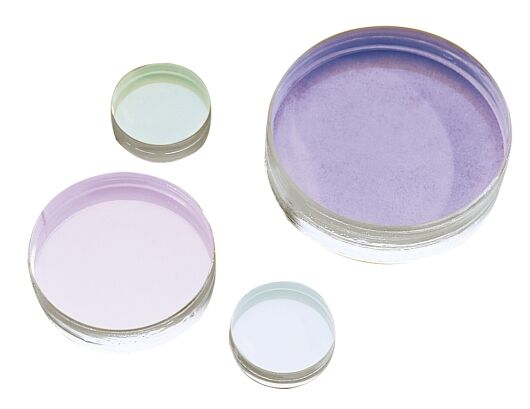
Different waveplates work best in different applications depending on environment and bandwidth. Courtesy of Newport Corporation.
2. Fundamental Concepts in Polarization Control
Polarization is a fundamental characteristic of light that describes the orientation of light waves’ oscillations. At its core, it can be understood through electromagnetic theory, which posits that light consists of perpendicular electric and magnetic fields oscillating as they propagate. Depending on the orientation and phase relationship between these fields, light can exhibit different types of polarization: linear, circular, or elliptical.
In linear polarization, the electric field oscillates in a single plane as the light wave moves forward. This is the simplest form of polarization and is often manipulated using polarizing filters, which allow only light waves oriented in a specific direction to pass through. Circular and elliptical polarizations are more complex, involving electric fields that rotate around the direction of propagation, creating a helical pattern. Circular polarization arises when the electric and magnetic fields oscillate with equal amplitude but with a phase difference of 90 degrees, resulting in either left-handed or right-handed polarization.
Wave optics principles provide a deeper understanding of how devices like waveplates can alter the polarization state of light. Waveplates, made from birefringent materials, introduce a phase shift between the orthogonal components of an incoming light wave, effectively changing its polarization. The manipulation of polarization through such devices is crucial in various applications, from enhancing the contrast in optical microscopy to securing communication channels in fiber optic networks.
Understanding and controlling light’s polarization state through these principles enables advancements in optical technology, emphasizing the importance of polarization in the broader context of electromagnetic and wave optics theories.
3. Waveplates
Waveplates are one of the most versatile and commonly used pieces of optical equipment. Researchers use waveplates to shift polarization from linear to circular, from circular to linear, or to rotate linear polarization angle. These devices are often used in combination with other components to optimize optical setups. Light will often shift phase as it reflects and moves throughout an optical system. Waveplates are therefore vital in “optical cleanup.” Below we cover how to choose the correct wave plate for your application based on the desired phase correction.
Material Science of Waveplates:
Waveplates leverage the unique properties of birefringent materials, where the refractive index differs along different crystallographic axes. Common materials include quartz, mica, and calcite, each chosen for specific wavelength and environmental stability requirements. Quartz, for instance, is widely used for its broad transparency range and durability, making it suitable for a variety of optical applications. The intrinsic birefringence of these materials enables the waveplate to introduce a controlled phase shift between orthogonal polarization components of light, altering its polarization state effectively.
Design and Manufacturing of Waveplates:
The design of waveplates involves precise control over thickness and orientation of the birefringent material, as these factors determine the phase delay imparted on the passing light. Manufacturing processes employ sophisticated cutting and polishing techniques to achieve the desired optical path difference with high accuracy. Recent technological advancements have introduced methods like ion beam milling and laser engraving to fabricate waveplates with complex patterns and functionalities, further expanding their application scope.
Application-specific Variants:
Waveplates are tailored for specific applications by adjusting their design and material properties. In ultrafast optics, for example, achromatic waveplates are used to maintain the polarization state of broadband pulses. These waveplates combine multiple birefringent materials to achieve a nearly constant phase delay across a wide wavelength range. Other specialized variants include zero-order waveplates for high-precision applications and multiple-order waveplates for more cost-sensitive uses, each offering unique benefits in terms of phase shift, bandwidth, and environmental resilience.
4. Multiple Order Waveplates
Multiple order wave plates create a large phase difference that is several times the light’s wavelength. These devices provide either quarter-wave or half-wave retardant. They are the least expensive of the plates, but it is important to note that multi-order plates are extremely sensitive to changes in wavelength and temperature. Researchers often use multi-order plates with monochromatic light (such as a laser) . For example, multi-order plates are extremely popular in laser cutting end etching, which greatly benefits from circular polarization.
5. Zero Order Plates
As the name implies, zero-order plates cause a much smaller phase shift- one that is ideally less than a fraction of a wavelength. Retardation for these devices is much more constant in temperature and wavelength changes, so they can be used in a wider range of applications than the multi-order. They are especially useful in experiments that cannot be executed in temperature controlled environments.
6. Achromatic Plates
Achromatic waveplates consist of two materials that effectively eliminate chromatic dispersion. These devices attain almost constant retardation across a wide wavelength range. This makes achromatic waveplates ideal in applications requiring a broad bandwidth such as spectroscopy or mode-locking.
7. Fiber Optic Polarizers
Fiber optic polarizers are designed to filter and transmit light of a specific polarization while blocking others, ensuring that only the desired state is maintained along the fiber. These devices exploit the principle of birefringence, similar to waveplates, but are integrated directly into the optical fiber. The core technology involves aligning the fiber’s intrinsic birefringence or adding stress-applying parts to create a highly polarized light path. Key technical specifications include the extinction ratio, which measures the device’s ability to extinguish unwanted polarization, and insertion loss, indicating the amount of signal loss caused by the polarizer.
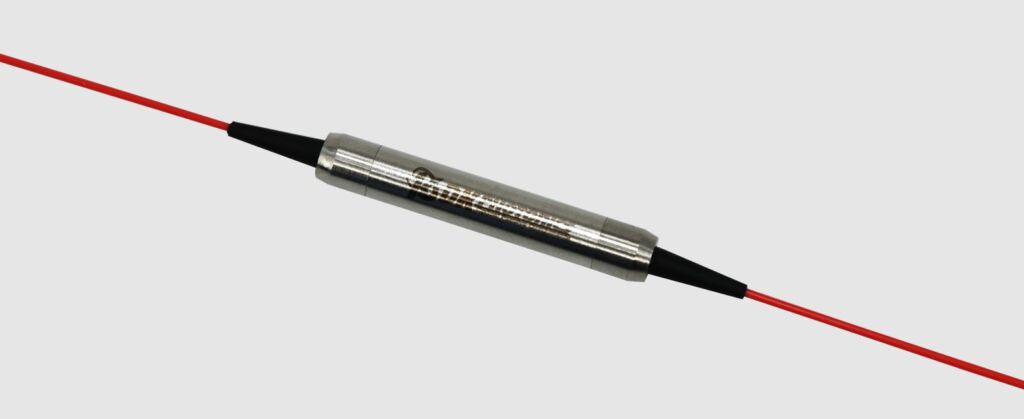
Fiber optic polarizers improve performance within fiber cables. They allow transmission of only one type of polarization. Courtesy of DK Photonics.
8. Polarization-Maintaining Fiber
Optical fibers are an extremely efficient light transmission devices, but they always exhibit some degree of birefringence. This causes changes in the polarization of transmitted light along the optical path. Researchers can utilize polarizer devices within these systems to create a polarization-maintaining fiber. These are specialty fibers with a strong built-in birefringence such that usual fiber disturbances do not shift polarization. Researchers frequently use the fibers in optical amplification, interferometry, and certain fiber lasers.
9. Optical Isolators
Working Mechanism: Optical isolators function based on the magneto-optic effect, which allows light to pass in one direction while blocking it in the opposite direction. This non-reciprocal behavior is typically achieved through the use of a Faraday rotator, a core element that utilizes a magneto-optic material (like garnet) placed within a magnetic field. As light passes through the rotator, its polarization plane is rotated due to the Faraday effect—a phenomenon where the polarization plane of light is rotated under the influence of a magnetic field along the direction of propagation. Paired with polarizers at the input and output, the device ensures that only light traveling in the desired direction can pass through, effectively isolating components from back reflections and feedback.
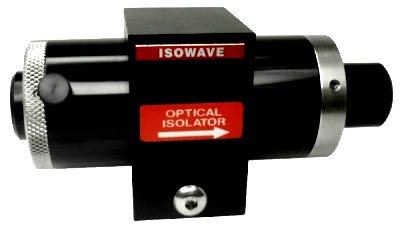
Optical isolators utilize polarization properties to block unwanted back-reflection during transmission. Courtesy of Deltronic Crystal.
Types and Differences: Optical isolators are categorized based on their design and operational wavelength. Faraday isolators, the most common type, are favored for their broadband performance and high isolation levels. They are widely used in laser systems to prevent destabilizing feedback. Another variant is the waveplate isolator, which relies on waveplates and polarizers to achieve isolation, typically used in narrowband applications. The choice between these types depends on factors such as the required isolation degree, bandwidth, and the specific application’s sensitivity to back reflections.
Challenges and Solutions:
One of the main challenges with optical isolators is their insertion loss, particularly in high-power laser systems where efficiency is critical. Research in this area focuses on optimizing materials and designs to minimize losses while maintaining high isolation. Thermal effects and polarization dependency also present challenges, especially in systems requiring stable performance over a broad range of temperatures and wavelengths. Advances in materials science, such as the development of temperature-independent magneto-optic materials and designs that compensate for thermal and polarization-induced variations, are among the solutions being explored to address these issues.
By understanding the principles, types, and challenges associated with optical isolators, researchers and engineers can better integrate these critical components into optical systems, enhancing performance and reliability across a range of applications.
10. Interferometers
Fiber optic interferometry is a technique in measuring sample properties and detecting changes in the system. This method uses superimposed waves to create an interference pattern and determine measurements of displacement, surface irregularities, and changes in refractive index. Interferometry is common in fields of astronomy, metrology, spectroscopy, and many branches of chemistry. Fiber optic interferometers often incorporate fiber optic isolators to ensure that light only travel in one direction and to prevent back-reflection. This allows the polarization devices maximum accuracy and precision.
11. Laser Resonators
Optical isolators can be useful components within a laser cavity. Laser quality and performance can often decay over time due to back-reflections into the laser cavity. Installing an optical isolator into the system allows a signal beam to streamline forward while keeping reflection from damaging the resonator. Isolators have shown to produce a more stable, more power efficient, and longer lasting laser. The devices are largely beneficial in systems for laser communications, transmission, and amplification.
12. Advanced Applications
Exploring advanced applications, polarization control emerges as a pivotal factor across diverse fields:
Quantum Computing: In quantum optics, polarization serves as a critical parameter for encoding quantum information. Quantum bits, or qubits, can be represented by the polarization states of photons, enabling the execution of quantum computations. This reliance on polarization necessitates precise control mechanisms to ensure the integrity of quantum states. Thus, polarization control not only facilitates the generation and manipulation of entangled photon pairs but also underpins the accuracy and reliability of quantum communication networks.
Telecommunications: Moving to telecommunications, polarization control significantly enhances fiber-optic data transmission. By managing polarization, systems can prevent signal degradation caused by polarization mode dispersion, a common issue in long-haul fiber networks. This control allows for the use of both polarization states to double the channel capacity, leading to more efficient and faster data transmission. Moreover, polarization-maintaining fibers ensure consistent signal quality over vast distances, crucial for the backbone of global communication networks.
Medical and Biomedical Applications: In the medical realm, polarization offers unique insights into tissue properties and structures. For instance, in optical coherence tomography (OCT), polarization-sensitive variations provide detailed images of tissue birefringence, aiding in the diagnosis of conditions like glaucoma. Furthermore, polarized light therapy uses specific polarization states to stimulate healing processes, demonstrating the therapeutic potential of polarization control. These applications underscore polarization’s versatility, extending its benefits from enhancing diagnostic accuracy to offering novel treatment modalities.
Across these sectors, polarization control stands out as a fundamental tool, shaping advancements in technology and medicine by enabling more precise, efficient, and innovative solutions.
13. Future Trends and Research Directions in Polarization Control
Looking ahead, the future of polarization control is marked by exciting technological innovations and research frontiers:
Emerging Technologies: One of the most promising areas involves the development of meta-materials and nanostructures that offer unprecedented control over light’s polarization. These materials can manipulate light in ways traditional optics cannot, opening up new possibilities in creating ultra-compact and efficient polarization devices. Such advancements hold transformative potential for industries ranging from telecommunications, where data capacity and transmission efficiency are paramount, to augmented and virtual reality, which demand precise light control for immersive experiences.
Research Frontiers: At the forefront of research in polarization optics are efforts to understand and exploit complex light-matter interactions at the quantum level. Scientists are exploring phenomena like quantum entanglement and its implications for polarization control, aiming to harness these effects for quantum computing and secure communication systems. Experimental work is pushing the boundaries of how polarization can be used to control light at the single-photon level, critical for the next generation of quantum technologies.
These emerging technologies and research efforts indicate a vibrant future for polarization control, promising to revolutionize how we manipulate light and its applications across a spectrum of fields. The ongoing exploration of these frontiers is poised to yield groundbreaking advancements, further embedding polarization control as a cornerstone of modern optical science and engineering.
14. Conclusion and Outlook
In conclusion, mastering polarization control is essential for the advancement of optical systems. The ability to manipulate light’s polarization state has profound implications across a wide array of applications, from enhancing the precision of scientific instruments to improving the efficiency of global communication networks. The versatility of polarization control devices, including waveplates, polarizers, and optical isolators, underscores their significance in refining optical performance and enabling new technological capabilities.
Looking forward, the future of these devices is promising. Innovations in materials science and nano-fabrication techniques are set to introduce more sophisticated and miniaturized devices, further integrating them into diverse technologies. As research pushes the boundaries of quantum optics and photonics, we can anticipate novel technologies that will revolutionize fields such as quantum computing, telecommunications, and medical diagnostics. The journey of understanding and harnessing the power of polarization is just beginning, with endless possibilities on the horizon.