Ultrafast optics is a very popular field for using short pulses to investigate phenomena that have remained a mystery with traditional optics. It has enabled investigation of fast reactions, high power applications, and has introduced new standards for optical metrology. While ultrafast optical systems can be built with fiber lasers and solid-state lasers, pulsed diode lasers offer the advantage of miniaturization, low cost, and monolithic designs that are all very attractive.
Pulsed diode lasers made from diode lasers are of two types: edge emitting and surface emitting. Vertical cavity surface emitting laser (VCSEL) is a type of surface emitting laser that is used frequently to make pulsed diode lasers. Pulsed diode lasers made from surface emitting VCSELs, can be used for fast optical serial communication such as fiber optic communications. Here, the pulses can be turned on and off to send data through the fiber cable.
Pulses made from diode lasers can also be used to measure frequency and time. The diode lasers used for this purpose are mode locked to give a pulse train. Since these pulse trains are equally spaced in time, one can use this as an optical clock. Meanwhile, if one looks at the pulse in the frequency domain, one sees that the frequencies present in the signal are also equally spaced. By letting an unknown signal interfere with the pulse train, one can then measure the unknown frequency. We will talk about this in more detailed a bit later in this article.
In this blog post we will learn the key design principles and architecture of a pulsed diode laser, as well as its applications in two important areas of science and technology: frequency combs and serial communication. First, we will study a key component that makes up a pulsed diode laser – the saturable absorber. Then we will look into the principles of pulse generation and get acquainted with the details of a typical setup.
Semiconductor Saturable Absorbers
Saturable absorbers are devices that absorb strongly at low intensities and are transparent at high intensities. They are the most important component in a passively mode-locked pulsed diode laser, so we will spend some time to discuss them in detail. Semiconductor based saturable absorbers are compact than designs from doped solid-state materials. A typical design structure is based on a Bragg reflector and a quantum well at either end of the reflector. The saturable absorption process happens in the quantum well. While there are many applications of saturable absorbers, this article only discusses its relevance with respect to pulsed diodes. The image below shows a cross section of such a semiconductor saturable absorber. The Bragg reflector is designing using alternating stacks of Ga and Al arsenide.
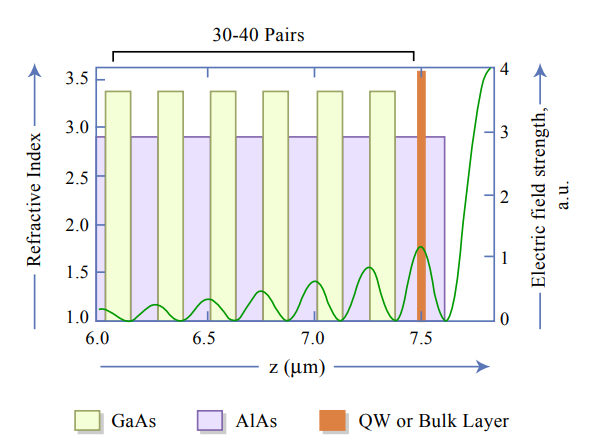
Saturable Absorber with Bragg reflector and Quantum well. Courtesy OCW.
A saturable absorber works by making electrons unable to reset to the initial state as fast as the number of incoming photons. In a semiconductor saturable absorber, the ground state is in the valence band and the excited state in the conduction band. If most of the carriers are in ground state, then incoming light can cause electrons to go to the excited state by absorption. However, if most of the electrons are in the excited state, incoming light cannot be absorbed. The light just passes through instead and the absorption is limited. The timescales for various processes must be looked into while determining if a semiconductor material will be good as a saturable absorber. The image below shows a sketch of various processes and their time scales within which a semiconductor interacts with incoming light.
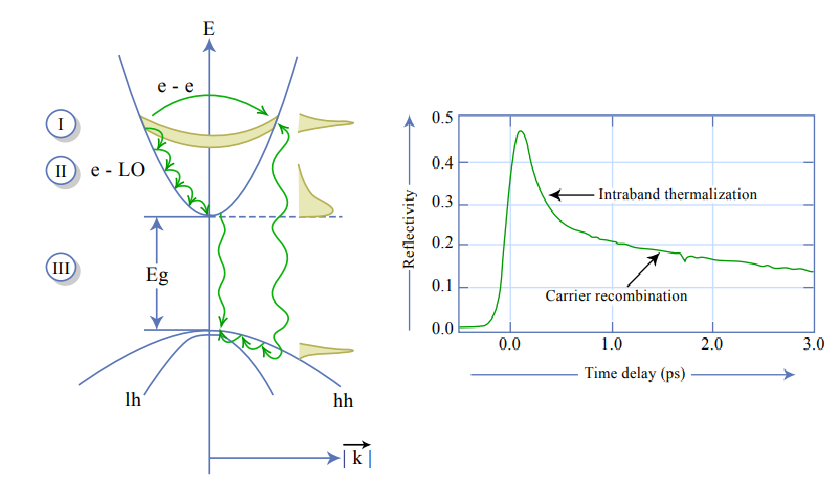
Saturable absorption: I) Photon absorption II) Thermalization process emitting heat, III) Recombination process. The figure on the right shows the carrier decay time scale. Saturable absorption is strong in I). Courtesy OCW.
The whole process is broken down into three steps: photon absorption (I), thermalization (II), and carrier recombination (III). During I, light absorption is maximum, and during II and III light absorption is minimum. This repeats over and over. Absorption is saturated in II and III. If steps II and III are long, the material is saturated easily. The use of saturable absorption in pulse generation comes from this ability to modulate its own absorption process just from the intensity of light.
Pulse Generation in Laser Diodes
Pulsed diodes that operate using the passively mode-locked scheme, typically have a gain section and a saturable absorber loss section. The saturable absorber introduces high losses in the cavity for small pulses, and low losses in the cavity for high intensity pulses. Thus, the high intensity pulses are favorably passed through the saturable absorber. If the gain for high intensities is sufficiently high, stable sustainable pulse trains can be obtained. Therefore from a random initial pulse train, a series of mode locked pulses can be obtained. The image below shows the power transmission and losses in a saturable absorber.
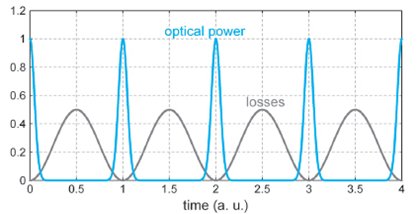
Transmission and losses in a saturable absorber over time. Courtesy SPIE.
Analysis in the frequency domain helps understand the various frequency components available in the pulse train. The job of the saturable absorber is to introduce a constant phase difference between the various modes available within the gain spectrum. This causes a pulse train in the time domain. A bigger gain bandwidth gives a smaller pulse width, and a smaller spacing between the modes gives a higher repetition rate. The image below shows the typical modes in a pulsed diode laser output spectrum.
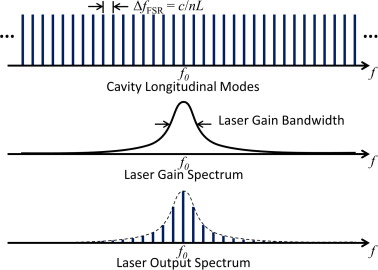
Observed frequencies in a pulsed diode laser.
A Typical Setup of a Pulsed Diode
Pulsed diodes have the gain and the saturable absorption sections integrated together as shown in the image below. The device on the right shows a monolithic mode-locked semiconductor laser diode. The gain section appears in the left, and the saturable absorption section modulated by a voltage appears in the right.
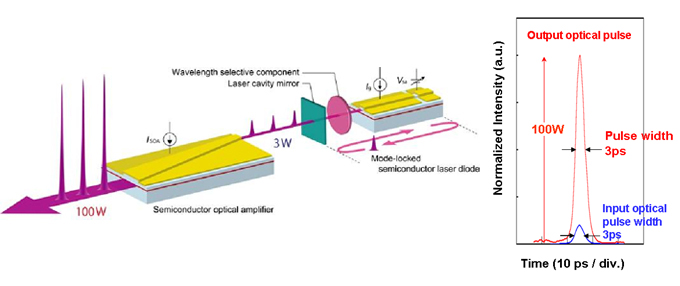
Pulsed semiconductor lasers with additional amplifiers to increase output power. Courtesy Phys.org.
The output parameters that are of interest in pulsed diodes are the pulse width, repetition frequency, and the bandwidth. Smaller cavities give a higher repetition rate, for this reason pulsed diodes can give repetition rates of many GHz. The typical powers of a pulsed laser diode are not as high as solid-state and fiber lasers for generating high power. However, a semiconductor optical amplifier can be used to enhance the pulse output to many watts.
Applications of Pulsed Diodes in Frequency Combs
Compact diode lasers can be used as a very precise frequency rulers for measuring optical frequencies. The pulse train has a number of equally spaced frequencies in the frequency domain which is called a frequency comb. Interference between the unknown input frequency and the closest frequency in the frequency comb causes a small beat frequency to occur which can be detected. The image below illustrates how a frequency comb can be used as a ruler to know the unknown input frequency.
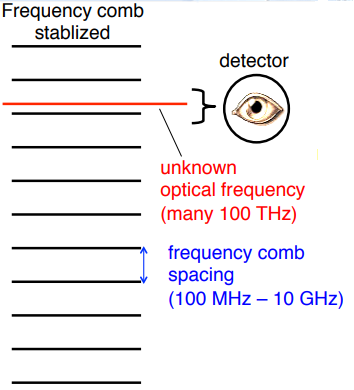
Frequency combs used as rulers to detect unknown frequency. The beat frequency is used to detect the unknown signal.
Using this method, very precise frequency combs can be obtained for quantum optical metrology. The precision of these devices can be further controlled by reducing variation in pulse spacing. This is done by a feedback mechanism where the output pulse jitter is reduced. Thus, the error in frequency spacing can be made very small.
Applications of Pulsed Diode Lasers in Serial Communication
Optical fiber communication using pulsed diode VCSELs is achieved in the ranges of tens of GHz. Pulsed VCSELs are modulated to send data down an optical fiber at around 1.55 um or 1.3 um. This has made long distance communication systems such as internet possible.
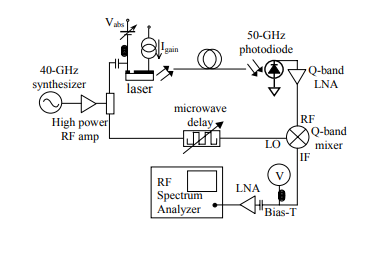
Pulsed laser used for high speed serial communication. Laser sends data, and the photodiode detects the data. Courtesy orbit.dtu
Pulsed diode VCSELs can be integrated seamlessly with electronics for a compact communication system. The circuit shows the full setup of such a communication system. The saturable absorber is modulated to send a pulse or turn it off. The modulated signal is to sent into a fiber cable which is detected by a high bandwidth photodiode to decode the information.
For pulsed applications, check out FindLight’s website for various pulsed diode lasers, and fiber coupled laser diodes for communication systems.