Nanotechnology continues to fascinate us with smaller and smaller devices. Engineers all around the world are constantly pushing and perfecting our manufacturing capabilities to fit more devices within a pin tip. One such device is a quantum dot which can be within just a few nanometers. For comparison, that is less than a thousandth of a human hair. They are so small that they share some of the properties of atoms. For this reason, they are referred to as artificial atoms at times.
Their unique properties continue to pave avenues for expanding the horizons of science and technology that were the bounds imposed by the macroscopic non-quantum world. One such application of quantum dos is in quantum dot (QD) lasers, which have proven to provide better energy conversion efficiencies than quantum well (QW) lasers.
In biology, quantum dots’ allow studying individual biomolecules, investigating their properties unclouded by the noise of an ensemble that is prevalent in traditional experimental techniques. This is enabled through funcionalization of quantum dots with biomolecules and harnessing quantum dots’ excellent emitting properties.
In this blog post we will become familiar with the structure and properties of quantum dots and look into their applications as green lasers and as single photon emitters.
Energy Structure of Quantum Wells and Dots
Size plays a crucial role in determining the energy levels that are distributed within a material. Dimensional size is a key parameter that determines the energy gaps of the materials. Thus by just making structures smaller or bigger, materials can be made to fluoresce in the visible or IR region. Quantum wells (semiconductor materials that are small only in one dimension) based on GaAs and InP have been used for this purpose to get surface emitting lasers for the red to infrared region. The variation in the number of energy levels with energy is referred to as the density of states (DOS). The following diagram illustrates how the reduction of size in one ore more dimensions affect the density of states:
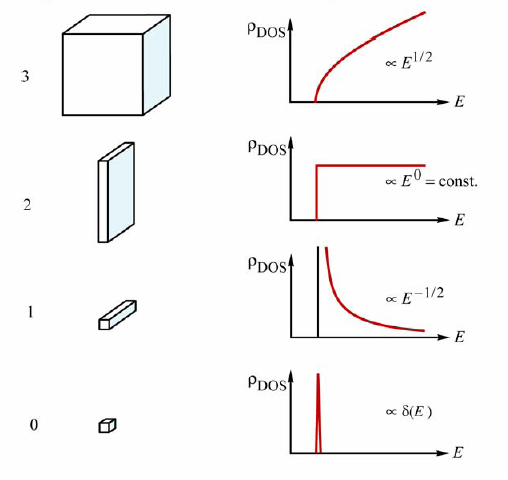
Density of states for structures of different dimensions.
Quantum dots are considered zero-dimensional materials that have discrete energy levels with strong peaks as we can see in the diagrams above. The property of having discrete strong peaks is very akin to energy levels seen in an atoms. We can see now why referring to these structures as artificial atoms is justified. By controlling the size of these objects, engineers have been able to synthesize quantum dots that emit in the blue, green or red part of the optical spectrum.
How to Synthesize Quantum Dots
There are two types of fabrication methods for quantum dots: top-down and bottom-up. Top-down methods start from imprinting a pattern on a bulk substrate and etching regions till the desired final structure is obtained. In contrast, bottom-up methods build the design from constituent atoms and molecules.
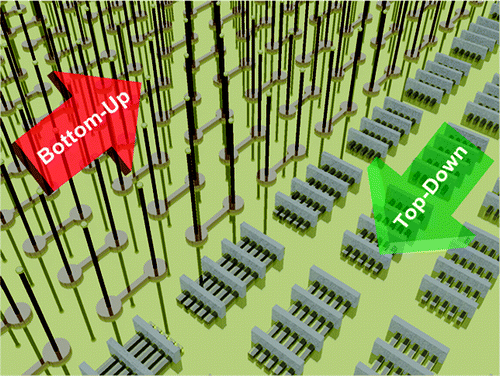
Two main fabrication methods: bottom-up and top-down.
Bottom-up methods are used to produce quantum dots as they are formed with better quality and show potential for mass manufacturing. Top-down methods are not able to obtain good surface smoothness using present lithographic techniques. Solution based bottom-up methods let the quantum dots form as colloids. The required size, uniformity and composition are obtained by controlling the temperature and material choices. Non-liquid bottom up methods exist with the quantum dots grown on top of a substrate with a large strain mismatch. The strain difference causes the deposited molecules on top to break up into small islands of quantum dots. This method is referred to as Stranski-Krastanov method for growing quantum dots.
Quantum Dot Lasers
Quantum lasers such as quantum well lasers can be made using molecular beam expitaxy (MBE) for obtaining very precise control of various layers. The MBE method can be combined with the middle layer grown in Stranski-Krastanov mode to get a layer of quantum dots. This layer can function as the active gain medium in quantum dot lasers.
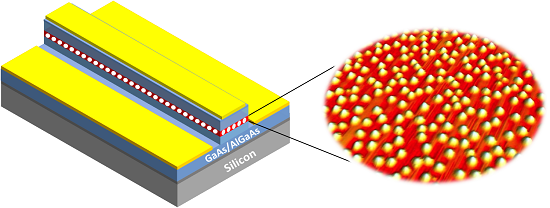
Surface emitting laser with a quantum dot layer as the active medium. Courtesy of LaserFocusWorld
Quantum dot lasers are better than quantum well lasers as they increase quantum efficiency and are more stable with temperature. One of the current problems that quantum wells face is the drift of threshold currents over time due to device heating. Since the quantum dots are only weakly coupled to the material vibrations (phonons), and the gain cross-section is 2-5 times higher than quantum wells, their wavelength varies less with temperature. Thus they are more stable with temperature variations. Additionally, higher gain can be obtained at lower threshold currents as shown below. This makes them more energy efficient than QWs as they draw less electrical power for the same light output.
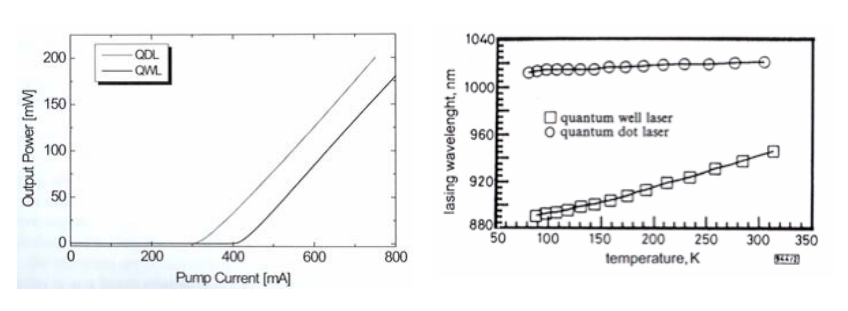
Comparison of output power (left) and temperature stability (right) for quantum dots and quantum wells. Courtesy of LecNotes
Green Quantum Dot Lasers
Quantum wells using nitride semiconductors are currently popular for light emission in the visible and UV region. These devices are more efficient in the blue region and not as efficient in the green region. This has resulted in the ‘green gap’, where efficient high power lasers in the green region are hard to design. Quantum dot lasers made from nitride semiconductors improve the emission efficiency of green light and can thus be used to generate high power green light outputs. Integrating green quantum dot lasers with efficient blue and red light lasers can make the next generation energy efficient visible lighting solutions possible.
Quantum Dot Applications in Biology
The discrete energy levels of quantum dots are useful to make single photon emitters. Since the linewidths of these energy levels are narrow, electrons can emit a single photon when they fall from the excited to the ground state. External light can be used to modulate the electronic state to produce a single photon on demand. Moreover quantum dots of various frequencies can be obtained just by varying the size.
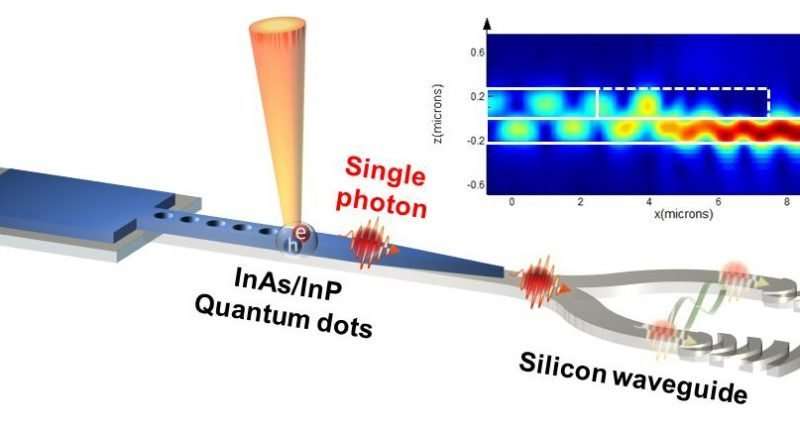
Single photon emitter for use in photonic circuits. Courtesy of semiconductor-today
Single photon emitters have been explored as device components for quantum communication. When integrated with photonic circuits, the single photons can be guided to a specific destination. For example, InP quantum dots emitting light in the IR region can be guided by silicon waveguides that have low losses in the IR region. These demonstrations, while still in the laboratories, can soon revolutionize optical communications we know today.
Did you know, FindLight has an extensive list of suppliers for such products as laser diodes, quantum well surface emitting lasers, and photon counters that you can use in your laboratories to make the next breakthrough in science and technology.