Nanowires are truly one-dimensional structures that are long in one direction and short on the lateral dimension. This dimensional reduction gives rise to interesting new phenomena such as quantized conductivity that can be utilized to make novel electronic devices and nano-channels. To employ them for engineering functional devices they often need to be assembled into large ensembles. Because of their elongated shape, side-by-side stacking is a natural geometry for achieving a high density of these nanoscopic objects.
Engineered correctly, semiconductor nanowires find applications in lasers and in high efficiency photodetectors. For instance, many nanowire photodetectors can be densely packed close to each other to increase spatial resolution in light detection. In laser applications, nanowire based lasers can exhibit higher repetition rates and shorter pulse durations than those built on simple planar semiconductor diodes.
Nanowires also find applications in frontiers of technology. In particular, scientists have been using them as mediums to transport qubits to develop functional quantum computing machines. In this blog post, we will look into the structure and fabrication of semiconductor nanowires with their uses in photodetectors, lasers, and quantum optics.
Shape and Structure of Nanowires
Quantum confinement in the lateral dimensions causes the electronic energy levels in the nanowire to depend on its size. Another very important result of lateral confinement is that there are only discrete modes available for conduction along the nanowire. The manifestation of this is in the step like voltage-current curve shape. In other words, because of the discrete electronic modes, if the voltage across the wire is increased gradually, the current flow increases in step-like fashion. This property can be gainfully employed to engineer highly sensitive devices that will manifest similar properties as their semiconductor rivals.
More complex nanowires are fabricated by creating modifications radially or axially. Radial modification deposits different layers along the length of the nanowire. In axial structures, the layers are deposited in concentric circles from the center around the nanowire. Axial structures are better suited for photodetectors as they have a higher area for light absorption. The image below shows the various nanowire structures.
Fabrication of Semiconductor Nanowire
There are two main deposition methods for nanowire fabrication: bottom-up than top-down. Under the bottom-up approach, the nanowires are grown on the substrate rather than being patterned and etched. Patterning techniques introduce a degree of surface roughness that makes surface recombination of carriers very high and structures to vary widely form one other. Bottom-up approach gives more uniform surface structures. The typical bottom-up method used for semiconductor nanowire fabrication is Vapor-Liquid-Solid (VLS) growth as shown in the image below.
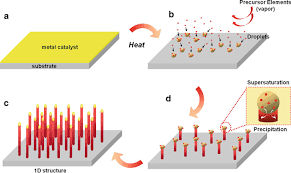
Deposition of nanowires by vapor-liquid-solid growth.
The crucial ingredient in VLS growth method is a metal catalyst such as gold deposited for synthesis. The gold catalyst can be initially patterned into small islands. Exposing the film to the semiconductor nanowire compounds causes deposition to occur selectively only at the gold island centers. This is because the vapor to solid transition of the semiconductor compounds is not favorable without the presence of gold catalyst under appropriate temperature and pressure. The nanowire length can be controlled by external conditions, and the resulting nanowire is highly uniform. The catalyzed growth is illustrated in the image below.
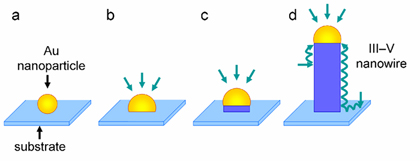
Steps in the catalyzed growth of a semiconductor nanowire. Photo courtesy of SPIE
The nanowires deposited are usually triangular or hexagonal based on the material choice and growth conditions. They can also withstand higher stress, an important quality that can be utilized to make nanowire based MEMS devices.
Nanowires in Photodetectors
Photoelectronic devices detect photons by converting light energy into electrical current. The incoming photon excites an electron to the conduction band of the detector. The structure is additionally designed to sweep away the excited charges giving rise to an electric current. In normal semiconductor diodes, the interaction area for the photon to current conversion is quite small. An array of nanowire detectors can change this by increasing the surface area for the conversion as shown in the design below.
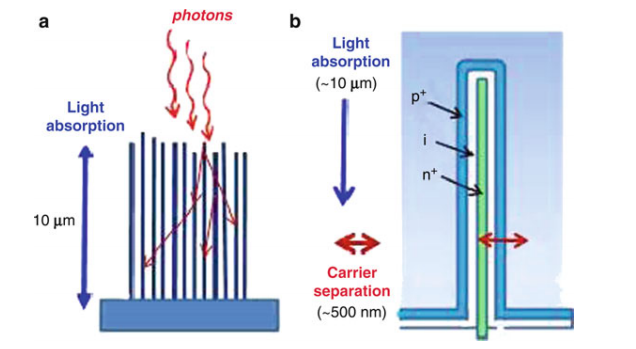
PIN photodetectors made using semiconductor nanowires. Courtesy Springer
Moreover, any reflected light can also be absorbed by the surrounding nanowires, thus further increasing the efficiency. Dense nanowire arrays have been used to make precise measurements of location for position sensors that take advantage of reabsorbing reflected light to increase efficiency in detection. Moreover, detected in this fashion photons can be processed in parallel for different nanowires to achieve much higher speeds of detection that those achieved by conventional CCDs. In conventional CCDs there is a fundamental speed limitation because the detected photons must be sequentially transferred between electron stacks for detection, which takes time.
Semiconductor Nanowire Lasers
In mode-locking, the high output repetition rate depends on the compactness of the laser cavity. Repetition rates from a pulsed semiconductor laser are usually within a hundred GHz. Nanolasers can achieve higher repetition rates at 200 GHz by reducing the cavity length to nanometer dimensions. The pulse width is also seen to be smaller than 3 ps.
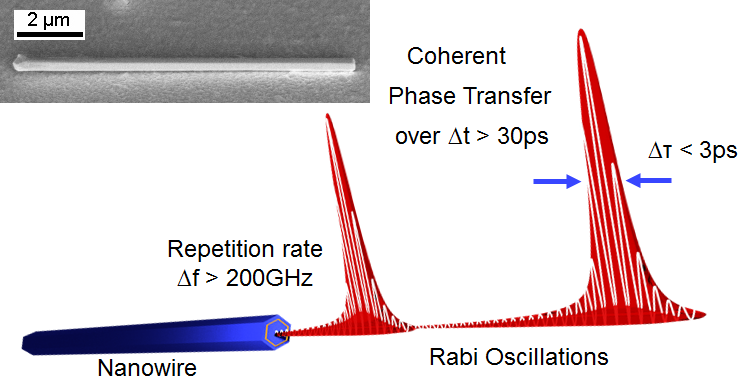
Semiconductor nanolasers with high repetition rates and shorter pulses. Courtesy Popflock
Semiconductor nanowire lasers consist of a wire made from higher refractive index material such as GaAs or other III-V compounds. The surrounding is a medium of lower refractive index, usually air. This causes reflections to occur at the two end facets as the light bounces back and forth. In this way, the nanowire sets up a Fabry-Perot cavity that can create light modes in the infrared region. The emission intensity is enhanced by Purcell’s effect if the nanowire is placed in a photonic crystal cavity. Research is being done to achieve other wavelengths in the infrared region close to 1.55 um for telecommunications. Applications include higher rate of data delivery, and nanowire laser arrays for parallel communication.
Nanowires that Transporting Qubits
Nanowires can support electron transport that are not disturbed by impurities or surface roughness. Because of this, coherent transport can be achieved under certain conditions. This can help any qubit information that an electron may carry to be preserved during transport. They act as mini cables for routing qubit information. Specific applications on this front include miniaturized quantum circuits for quantum computing that can generate, modulate, and transport qubits all in one chip.
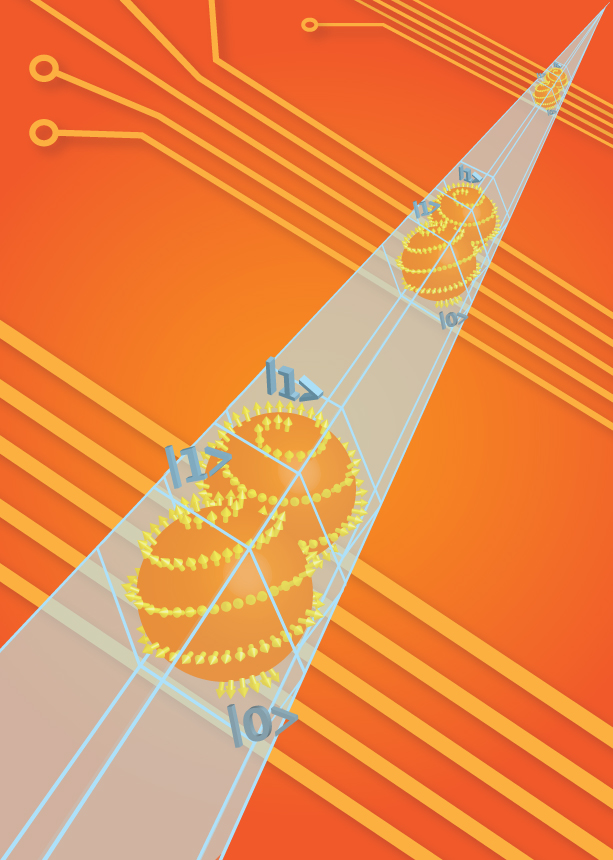
Nanowires for transporting qubits. Courtesy Nature
Did you know, FindLight’s vast collection of products for photodetectors, pin diodes, and CCD devices can used in your designs for detection and measuring light intensity.