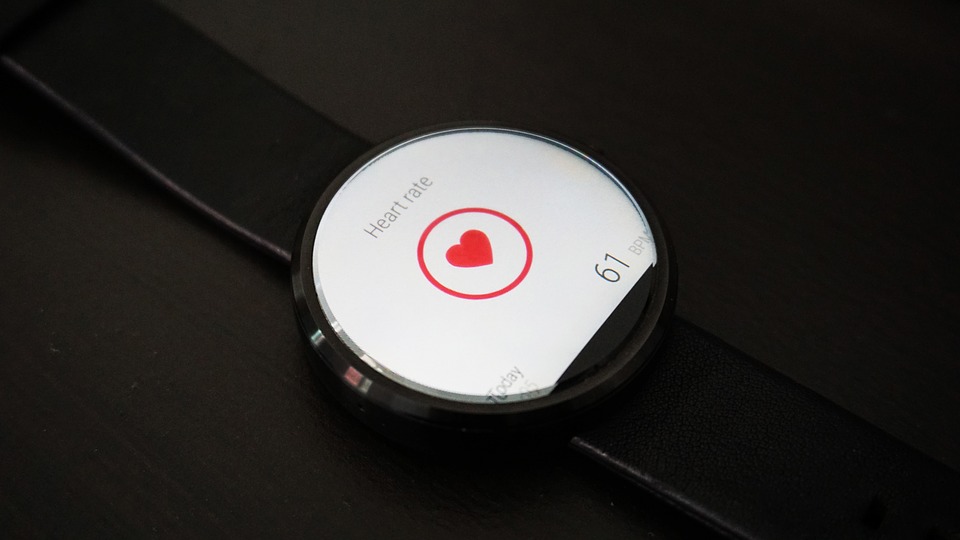
Non-invasive, wearable devices for the measurement of human vitals are gaining popularity in the healthcare field. Often times, these smart wearables are based on the use of optics. Developments in skin-like optical devices would allow for more sensitive and accurate measurements of vitals as the device conforms to the shape of the skin. Image courtesy of Pixabay.
Researchers from Tsinghua University in Beijing, China, recently published their findings on using the Monte Carlo method during the design of skin-like optical devices. The Monte Carlo method allows for the simulation of light being transmitted through tissue, including tissue which has been deformed.
The Benefits of Skin-Like Optical Devices
Optic-based measurement devices are widely used in healthcare for the measurement of vital signs as they are non-invasive. These sensitive optical devices rely on the emission and reception of light. For example, optical devices can measure blood oxygen saturation by detecting the absorption of light within blood vessels and surrounding tissues.
Skin-like optical devices are devices that can be mounted on human skin; they deform with the shape of the skin. The material used for skin-like devices is flexible and stretchable. In comparison, most optical devices are often rigid and cannot conform to irregular shapes. Skin-like devices are beneficial due to the potential for increased sensitivity and decrease in error. However, challenges exist in the use of these optical devices. The deformation of an optical device could change the optical path. Additionally, measurements taken of deformed skin could vary with those taken of normal skin, as deformation causes blood vessels and tissues to redistribute due to stress. These challenges motivated the Tsinghua University team to find a relationship between light signals and the deformation of skin and skin-like devices.
What is the Monte Carlo method?
The Monte Carlo method, or the probability simulation, is a category of computational algorithms. It allows for the simulation of systems with multiple coupled degrees of freedom for optimization problems. Generally, the Monte Carlo method is used to model the probability of various outcomes in a process or system that is difficult to predict due to multiple variables.
In the case of the optimization of optical device design, a Monte Carlo simulation can predict how light will be transmitted through human tissue with a variety of changing parameters. These parameters describe both the incident light, as well as the blood vessels found in the tissue.
Experimental Research & Findings
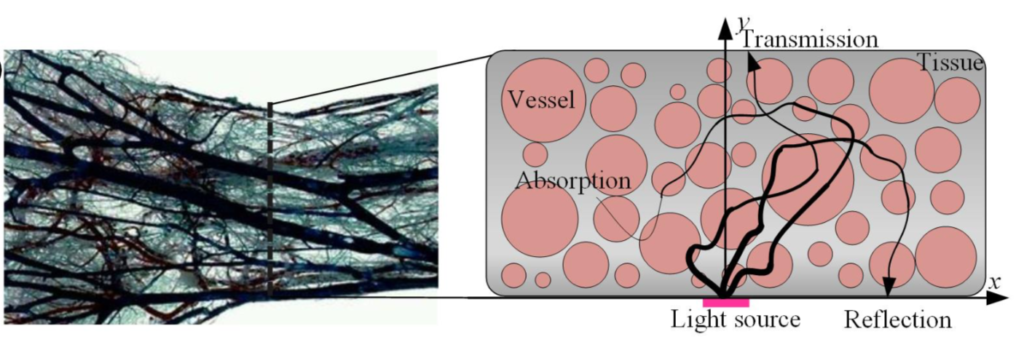
The figure above shows the schematic diagram of the 2D model used. Pictured on the left is a vascular casting of a human wrist. Pictured on the right is the simulated model. Image courtesy of Biomedical Optics Express.
In the experiment done by the research team, a 2D model was created to simulate a cross-sectional area of human tissue. This Monte Carlo simulation investigated excited light as it exited the human tissue. Excited light included both reflected and transmitted light. Both forms of light carry information about the patient’s vital signs following interaction with tissues and blood vessels. Blood vessels, which varied in diameter, density, and range, were randomly generated within this cross-sectional area. They were varied to reflect deformation of the skin. Parameters related to the incident light source were varied to reflect deformation of the optical device. Table 1, shown below, details the constants and variables in the Monte Carlo simulation during experimental research.
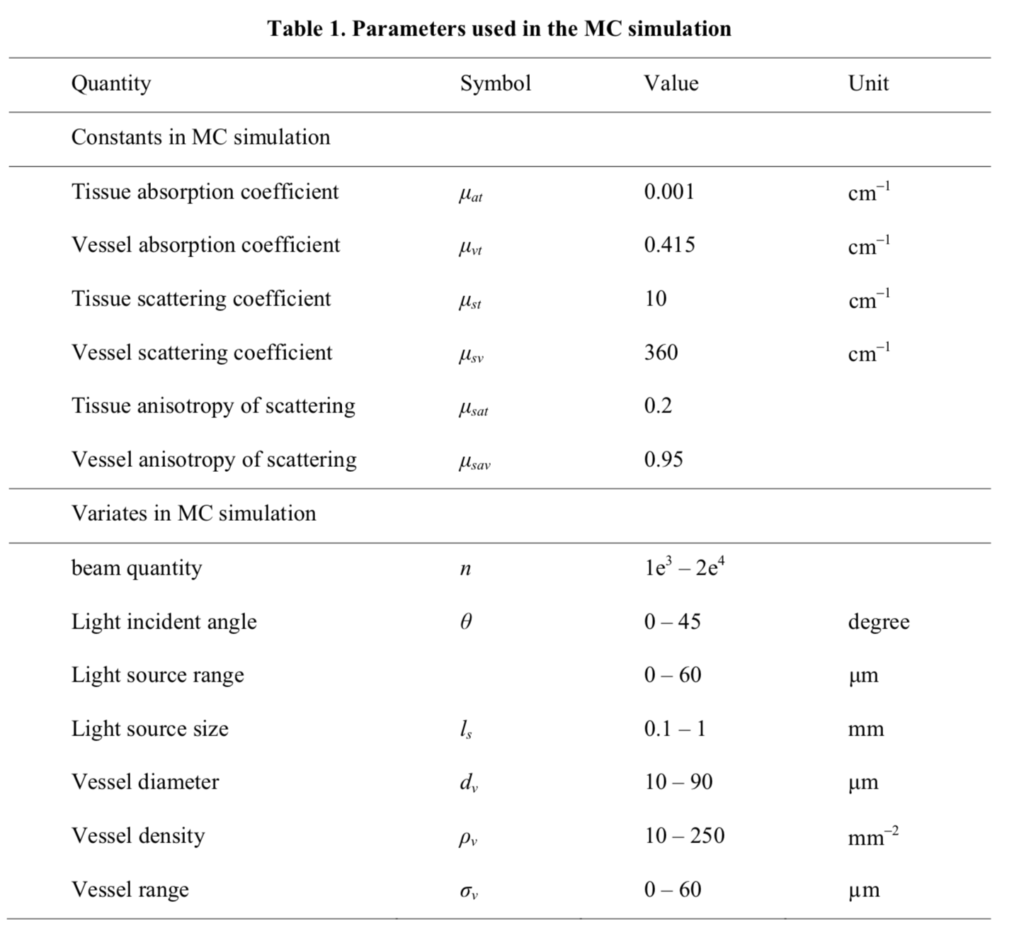
Table 1 shows all the parameters of the Monte Carlo simulation. Some parameters were held constant, others were varied. Courtesy of Biomedical Optics Express.
At the conclusion of the experiment, it was determined that blood vessel diameter and density, which change as a result of stress on the tissue being measured, do affect the characteristics of the light exiting the tissue. The relationship between the state of the blood vessels and excited light is nonlinear. When simulating deformation of the skin-like optical device, it was concluded that exiting light was independent of the light source’s size. However, the intensity of the exiting light was proportional to the intensity of the light source.
These discoveries and relationships found should be used in the future when designing skin-like optical devices.
Future Possibilities
Using data obtained from their experiment, the researchers were able to design and manufacture a rudimentary skin-like optical device. It was composed of two LEDs and a photodetector, and would be used to measure photoplethysmogram (PPG) signals, which detect blood volume changes. After initial testing, the research team concluded that their device was capable of detecting these PPG signals, both when the skin was not deformed and when it was deformed.
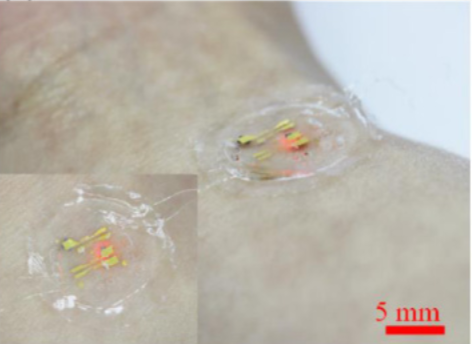
The figure above shows the skin-like optical device fabricated by the research team when placed on the skin. The inset shows the device from another angle. Image courtesy of Biomedical Optics Express.
Findings from this experiment would allow for improved skin-like optical devices to be designed. Smart wearables, which are growing in popularity, would certainly benefit from the addition of optical device technology and the accuracy that accompanies such devices. Additionally, low-cost, robust devices could be created for accurate vital measurements in low-resource settings. Their small size and flexible materials are less prone to destruction during travel or usage. Therefore, they are better candidates for low-resource settings. Finally, the high levels of accuracy associated with optical devices would be well appreciated in all forms of healthcare settings.
To read more about the use of the Monte Carlo method in the optimization of skin-like optical device design, the paper can be read here.