In this technical guide on streak cameras we provide a deep dive into the operational principles, advancements, and practical applications of streak cameras. Whether you’re an engineer, a researcher, or a tech enthusiast, this comprehensive guide will equip you with the knowledge to leverage this cutting-edge technology in various scientific and industrial settings.
Table of Contents:
- Introduction
- Historical Development
- Fundamental Principles
- Types of Streak Cameras
- Measuring Ultrafast Phenomena
- Advanced Technologies in Streak Cameras
- Applications of Streak Cameras
- Practical Considerations
- Future Directions
- Conclusion
- Appendices
- References
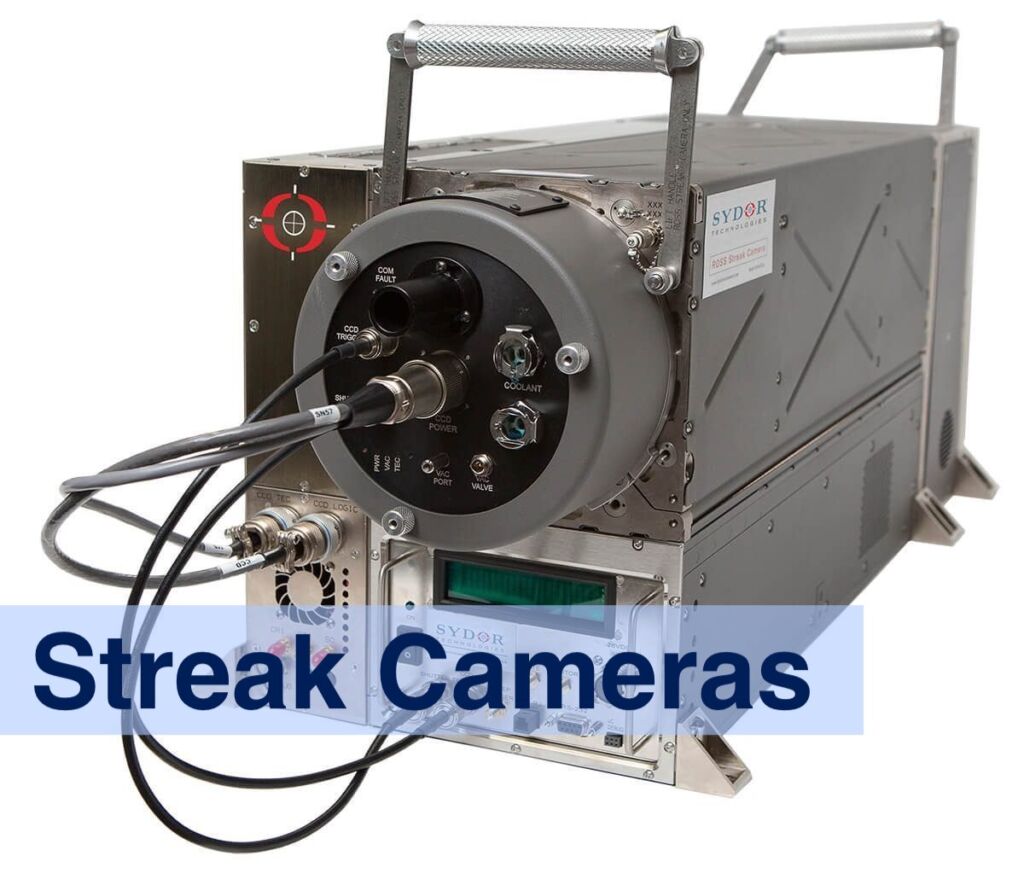
Featured: A high-precision streak camera, the cornerstone of ultrafast optical measurements. Image courtesy of Sydor Technologies.
1. Introduction to Streak Cameras
Streak cameras are pivotal in the field of high-speed optical research, where capturing fleeting light phenomena is crucial. These sophisticated devices translate temporal information into spatial data, allowing precise measurement of light intensity variations over extremely short durations. Engineers and researchers rely on streak cameras to analyze events ranging from femtoseconds to nanoseconds, a capability central to advancements in fields such as laser physics, plasma diagnostics, and biomedical imaging.
The operational principle hinges on converting photons into electrons via a photocathode. These electrons are then deflected and captured on a screen, creating a ‘streak’ that visually represents the intensity profile over time. This process not only supports the study of light’s behavior at near-light speeds but also facilitates the development of technologies like LIDAR and time-resolved spectroscopy.
Understanding streak cameras’ roles and mechanics is fundamental for those engaged in the cutting-edge of photonics and related sciences. In this guide we examine their historical evolution, fundamental principles, types, and applications, offering a comprehensive look at both the current state and future potential of this critical technology.
2. Historical Development
The genesis of streak camera technology dates back to the early 20th century, evolving from rudimentary imaging techniques to sophisticated systems capable of capturing light in motion at picosecond intervals. Initially developed to analyze the temporal dynamics of visible light, these devices have expanded their utility across various electromagnetic spectra, including ultraviolet and X-ray.
Key milestones in streak camera development include the introduction of the photocathode, which significantly enhanced sensitivity and temporal resolution. In the mid-20th century, the transition from mechanical to optoelectronic systems marked a pivotal advance, eliminating the limitations imposed by moving parts and substantially increasing the speed and accuracy of measurements.
The 1990s saw a leap in technology with the integration of charge-coupled devices (CCDs), allowing for digital recording and processing of streak images. This integration paved the way for more complex and reliable data analysis, crucial for applications in fast optical shutters and scientific cameras used in high-energy physics.
More recently, advancements in micro-channel plate technology have further refined the temporal resolution, enabling streak cameras to resolve events down to the femtosecond range. This progress not only underscores the rapid pace of innovation within the field but also enhances the capabilities of researchers to observe and manipulate physical phenomena at the quantum level.
As we continue to push the boundaries of what we can observe and measure, the historical development of streak cameras serves as a testament to the relentless pursuit of precision and understanding in scientific inquiry.
3. Fundamental Principles
Streak cameras operate by converting temporal data into spatial images, capturing the fleeting dynamics of light at incredibly short time scales. This section outlines the core principles and components that allow streak cameras to perform with such precision.
Photocathode: The heart of a streak camera is its photocathode, where photons of light are converted into electrons through the photoelectric effect. The efficiency of this conversion is critical as it determines the camera’s sensitivity and overall performance. Materials typically used for photocathodes vary based on the wavelength of light being analyzed, with some optimized for ultraviolet light, while others are suited for visible or infrared spectra.
Deflection Plates: Once electrons are emitted from the photocathode, they are directed towards the deflection plates. These plates apply an electric field perpendicular to the path of the electrons, causing them to deflect horizontally. The timing and magnitude of the voltage applied across these plates are precisely controlled to ensure that the position at which the electrons hit the detection screen corresponds accurately to the time at which the photons struck the photocathode.
Micro-channel Plate (MCP): The MCP is an essential component for amplifying the number of electrons. This plate consists of numerous microscopic channels that operate as independent photomultiplier tubes. When electrons pass through these channels, secondary electrons are emitted through a cascade effect, significantly increasing the electron count and thereby enhancing the image’s brightness and contrast.
Phosphor Screen: At the end of their journey, the electrons impact the phosphor screen, converting back into visible light. This screen captures the spatially distributed electron impact as a luminous image, known as the streak image. The brightness and color of the phosphor determine the quality of the final image output.
Photoelectric Effect: Fundamental to the operation of streak cameras, the photoelectric effect is the phenomenon by which electrons are emitted from materials (typically metals and semiconductors) when they absorb energy from light. In streak cameras, this effect is finely tuned to ensure that the electron emission is instantaneous and proportional to the light intensity, allowing for precise temporal resolution.
Together, these components form a complex but highly effective system for studying phenomena on scales as short as a few femtoseconds, making streak cameras indispensable tools in scientific research where time resolution is critical.
4. Types of Streak Cameras
Streak cameras are broadly categorized into mechanical and optoelectronic types, each with unique designs and capabilities tailored to specific applications.
4.1. Mechanical Streak Cameras
Mechanical streak cameras were among the first types developed and primarily use a rotating mirror or a moving slit system to deflect the incoming light beam across a photographic plate or sensor. The design relies on physical movement to achieve the temporal sweep necessary for capturing light intensity over time.
Limitations:
- Speed: The maximum deflection speed is constrained by the mechanical parts’ physical limitations, which significantly caps the temporal resolution.
- Complexity: The mechanical nature requires frequent maintenance and calibration to ensure accuracy, adding to operational complexities.
- Durability: Mechanical wear and tear over time can affect performance and reliability.
4.2. Optoelectronic Streak Cameras
Optoelectronic streak cameras represent a significant advancement over their mechanical counterparts. These devices utilize a photocathode to convert the light into an electron image, which is then deflected by an electric field within a vacuum tube, similar to how an old cathode-ray tube display works.
Differences from Mechanical Types:
- No moving parts: Optoelectronic streak cameras do not rely on mechanical movement, which eliminates many of the limitations associated with wear and maintenance.
- Faster operation: The deflection of electrons by electric fields allows for much faster operation speeds, achieving temporal resolutions in the femtosecond range.
Benefits:
- Higher speed and resolution: The use of electric fields to deflect electrons allows these cameras to operate at speeds that can capture ultrafast phenomena, making them ideal for advanced scientific research.
- Greater reliability: With fewer moving parts, optoelectronic streak cameras are more durable and require less maintenance.
- Versatility: They can be used in a wider range of applications, from laser diagnostics to plasma physics, thanks to their ability to quickly and accurately measure light across different environments.
The development of optoelectronic streak cameras has largely supplanted mechanical models in most advanced applications, thanks to their superior speed, resolution, and reliability.
5. Measuring Ultrafast Phenomena
Streak cameras excel in capturing ultrafast phenomena by translating variations in light intensity over infinitesimally short intervals into spatial data. In this section we look into the process through which streak cameras achieve this feat and delve into the significance of temporal resolution in unraveling rapid events.
Principle of Operation: Streak cameras operate on the fundamental principle of converting temporal information into spatial data. When a pulse of light strikes the photocathode, it liberates electrons through the photoelectric effect. These electrons are then accelerated and deflected by precisely controlled electric fields, causing them to sweep across a detection screen. The resulting spatial distribution of electrons, known as the streak image, corresponds directly to the intensity profile of the incident light pulse over time.
Temporal Resolution: Temporal resolution, the ability to discern changes in light intensity with extreme precision, is a defining characteristic of streak cameras. It dictates the shortest time interval over which variations in light intensity can be accurately measured. Achieving high temporal resolution is paramount in capturing fleeting phenomena such as femtosecond laser pulses or molecular dynamics.
Significance: The significance of temporal resolution lies in its capacity to reveal the underlying dynamics of ultrafast processes. By accurately measuring the duration and intensity of light pulses, streak cameras enable researchers to investigate phenomena ranging from chemical reactions to semiconductor dynamics with unparalleled precision. Moreover, high temporal resolution is indispensable in fields like laser physics and plasma diagnostics, where understanding transient events is crucial for advancing scientific knowledge and technological innovation.
Understanding the intricacies of streak camera operation and the critical role of temporal resolution is essential for researchers and engineers engaged in high-speed optical research. Mastering these principles helps scientists gain new insights into fundamental behaviors of matter and light.
6. Advanced Technologies in Streak Cameras
Recent breakthroughs in streak camera technology continue to push the limits of how quickly and accurately we can observe ultrafast phenomena. Innovators in the field are constantly enhancing the components and functionalities to capture events at the edge of time resolution.
6.1. Enter Femtosecond and Attosecond Cameras
A key development is the advent of femtosecond and attosecond streak cameras. These devices are groundbreaking because they allow scientists to track and measure light phenomena that occur in less than a trillionth of a second. Femtosecond cameras capture details of chemical reactions as they happen, while attosecond cameras delve into electron movements within atoms, a scale that was once deemed unobservable.
6.2. Boosting Temporal Resolution
Improvements in temporal resolution are at the heart of these advancements. Engineers have refined the electronics and the electromagnetic systems within cameras, allowing for more precise deflections of the electron beams. This refinement means that the cameras can now produce images that resolve time intervals down to a few attoseconds.
6.3. Enhancing Sensitivity
Alongside better resolution, enhanced sensitivity has been a focus. Modern streak cameras feature improved photocathodes that provide greater electron yields from incoming photons. This enhancement ensures that even events with very low light levels are captured clearly, increasing the range of experiments that can benefit from streak camera technology.
6.4. Implications for Research and Industry
These technological leaps not only expand our fundamental understanding of light and matter but also pave the way for innovations in fields like photonics, quantum computing, and medical imaging. As streak cameras become more sensitive and faster, they open up possibilities for new types of experiments and diagnostics that were previously beyond reach.
7. Applications of Streak Cameras
Streak cameras play a crucial role in a variety of scientific and industrial applications. Their ability to capture ultrafast events makes them indispensable in fields ranging from spectroscopy to photonics research.
7.1. Time-Resolved Spectroscopy
Time-resolved spectroscopy relies heavily on streak cameras to observe the dynamic processes within molecular and atomic systems. By measuring how light interacts with materials over extremely short timescales, researchers can understand phenomena such as energy transfer, chemical reactions, and electron dynamics. This insight is vital for developing new materials and enhancing our understanding of chemical processes.
7.2. LIDAR Technology
In LIDAR (Light Detection and Ranging) systems, streak cameras are essential for measuring the time it takes light to return after hitting an object, thus determining distances with high precision. This technology is fundamental in autonomous vehicle navigation, geology, and atmospheric research. The fast response time of streak cameras allows for the collection of high-resolution, real-time data from environments.
7.3. Laser-Induced Fluorescence Spectroscopy
Streak cameras also find applications in laser-induced fluorescence spectroscopy, where they monitor the fluorescence emitted by a substance when excited by a laser. This method is particularly useful in biological and medical diagnostics, providing insights into cellular functions and the molecular composition of tissues without invasive procedures.
7.4. Plasma Physics Diagnostics
In plasma physics, streak cameras are invaluable for diagnosing plasma behavior in fusion reactors and other plasma-based devices. They help scientists measure changes in light emission from plasma, enabling the study of plasma stability, density, and temperature changes over time. Such diagnostics are critical for advancing nuclear fusion research and other applications involving high-energy plasmas.
7.5. High-Speed Photography and Photonics Research
Finally, in the field of high-speed photography, streak cameras capture rapid phenomena that are invisible to the naked eye, such as shock waves and fluid dynamics, at speeds that can be visualized and analyzed later. In photonics, these cameras facilitate the study of light behavior at speeds and resolutions necessary for developing next-generation optical devices and systems.
Each of these applications demonstrates the versatility and critical importance of streak cameras in modern science and technology, leveraging their unique ability to measure and visualize events at unprecedented speeds and resolutions.
8. Practical Considerations
When integrating and operating streak cameras, engineers and researchers must consider several practical aspects to ensure optimal performance and accuracy. In this section, we cover selection criteria, maintenance, and common troubleshooting tips.
8.1. Selection Criteria for Specific Applications
Choosing the right streak camera hinges on understanding the specific needs of your application. Key factors include temporal resolution, sensitivity, and the spectral range. For instance, applications requiring ultrafast measurement, such as observing electron dynamics, necessitate cameras with femtosecond to attosecond resolution. Similarly, applications in low-light conditions will benefit from cameras with enhanced sensitivity and efficient photocathodes.
8.2. Maintenance and Calibration
Regular maintenance and calibration are vital to maintaining the streak camera’s performance. This typically involves cleaning the photocathode and checking the alignment of optical components to prevent image distortion. Calibration should be performed periodically to ensure the accuracy of time measurements, especially after the system has been moved or components have been replaced. Using standard calibration sources, like pulsed lasers, helps verify the camera’s timing and sensitivity settings.
8.3. Common Pitfalls and Troubleshooting Tips
Users often encounter issues such as signal degradation or synchronization problems. To mitigate these, ensure that the camera’s internal timing is precisely synchronized with the experimental setup. Signal degradation can often be addressed by checking and optimizing the electron path within the camera, ensuring that no external magnetic fields are interfering with the electron trajectories. Regularly reviewing and adjusting the deflection settings and voltage levels can also prevent performance lapses.
Furthermore, it’s crucial to understand the limitations of your device. Overloading the camera with too intense a light source can damage the photocathode, while underutilizing it can lead to suboptimal data due to noise levels. Always adjust the input light levels to match the camera’s operational range.
By considering these practical aspects, users can significantly enhance the functionality and longevity of their streak cameras, leading to more reliable and accurate data collection in their respective applications.
9. Future Directions
Streak camera technology is evolving rapidly, ushering in new possibilities across various scientific and industrial domains. This section highlights emerging trends, potential applications, and how integration with other technologies might shape the future of high-speed imaging.
9.1. Emerging Trends and Technologies
Developers are currently enhancing streak cameras with artificial intelligence and machine learning algorithms to automate data analysis and improve accuracy. These technologies enable the cameras to learn from the data they collect, optimizing settings for different experimental conditions automatically. Additionally, advances in materials science are producing more sensitive photocathodes, allowing for better performance in low-light conditions.
9.2. Potential New Applications and Markets
As streak cameras become more versatile and easier to use, they are finding new applications in fields such as environmental monitoring and security. For example, high-speed cameras can now monitor the dispersion of pollutants in the atmosphere or assist in the analysis of thermal and chemical signatures for security purposes. The medical field also shows promise, particularly in the study of neural activities and fast biological processes.
9.3. Integration with Other High-Speed Imaging Technologies
Streak cameras are increasingly integrating with other high-speed imaging systems like time-resolved fluorescence and multi-photon microscopy to provide a more comprehensive analysis of fast phenomena. This integration enables simultaneous spatial and temporal resolution at unprecedented levels, opening doors to previously unexplored areas of research.
Moreover, the potential to combine these systems with computational imaging techniques stands to revolutionize how we visualize processes at the quantum and cellular levels. By harnessing these integrated tools, researchers can capture a fuller picture of dynamic events, driving forward innovations in both science and technology sectors.
These developments not only broaden the scope of current applications but also promise to unlock new markets and opportunities, making streak cameras indispensable tools in the ongoing quest to understand and manipulate the fastest processes known in nature.
10. Conclusion
Understanding and utilizing streak cameras is crucial for capturing and analyzing phenomena that occur in mere femtoseconds. These devices provide unparalleled insights into the fast-paced dynamics of light, essential for advancements in scientific research and practical applications.
Streak cameras have evolved from basic mechanical devices to sophisticated digital systems that offer high resolution and rapid data processing. This evolution demonstrates the technology’s vital role in fields as diverse as physics, chemistry, and biomedical engineering.
Looking ahead, the potential of streak cameras continues to expand with advancements in technology and integration. As they become more sensitive and user-friendly, their use will likely extend beyond traditional laboratories to new industries and everyday applications.
In conclusion, the journey of streak cameras from niche scientific instruments to versatile tools highlights their growing significance. As we push the boundaries of what is measurable, streak cameras will play a pivotal role in unveiling the next layers of understanding in fast-moving scientific phenomena.
11. Appendices
This section provides additional resources to enhance your understanding and utilization of streak cameras. It includes a glossary of common terms, comparison tables of various models, and a comprehensive list of suppliers along with their product offerings.
Glossary of Terms
- Photocathode: A component that emits electrons when exposed to light, initiating the streak camera’s imaging process.
- Temporal Resolution: The smallest time interval that the camera can measure, typically expressed in femtoseconds or picoseconds.
- Electron Deflection: The process by which electrons are redirected by electric fields within the streak camera to form an image.
- Phosphor Screen: The surface inside the camera where electrons are converted back into visible light to create the final streak image.
- Micro-channel Plate (MCP): A device used to amplify the number of electrons, enhancing the image’s brightness and contrast.
List of Suppliers and Their Offerings
- Hamamatsu Photonics: Offers a range of streak cameras with varying temporal resolutions suitable for both scientific research and industrial applications.
- Sydor Technologies: Provides streak cameras with high temporal resolution and robust software support for data analysis.
- Photonis: Known for their innovative streak tube designs that offer high sensitivity and fast response times.
- FindLight: Our marketplace features a dedicated category of streak cameras, where you can find models from leading suppliers worldwide
12. References
This section compiles key references that have informed the content and conclusions of this article on streak cameras. It includes academic papers, patents, and industry reports that provide foundational knowledge and cutting-edge insights into the technology and its applications.
Academic Papers
- Reiser, M., & Faber, S. C. (1997). Recent and future advances in high-speed imaging. European Radiology, 7(Suppl 5), 166-173. https://doi.org/10.1007/pl00006888
- Kassier, G. H., Haupt, K., Erasmus, N., Rohwer, E. G., von Bergmann, H. M., Schwoerer, H., Coelho, S. M. M., & Auret, F. D. (2010). A compact streak camera for 150 fs time resolved measurement of bright pulses in ultrafast electron diffraction. Review of Scientific Instruments, 81(10), 105103. https://doi.org/10.1063/1.3489118
- Mondal, J., Maity, N. C., & Biswas, R. (2023). Detection of ultrafast solvent dynamics employing a streak camera. Journal of Chemical Sciences, 135, Article 84.
- Li, Z., Zgadzaj, R., Wang, X., Reed, S., Dong, P., & Downer, M. C. (2010). Frequency-domain streak camera for ultrafast imaging of evolving light-velocity objects. Optics Letters, 35(24), 4087-4089. https://doi.org/10.1364/OL.35.004087
- Wang, P., Liang, J., & Wang, L. V. (2020). Single-shot ultrafast imaging attaining 70 trillion frames per second. Nature Communications, 11, Article 2091. https://doi.org/10.1038/s41467-020-15745-4
Patents
- Wang, L., Liang, J., Zhu, L., & Ma, C. (2019). Multiple-view compressed-sensing ultrafast photography (MV-CUP). US Patent US10473916B2. Washington University in St. Louis. Retrieved from https://patents.google.com/patent/US10473916B2/en
- Tsuchiya, Y. (1990). Electro-optical streak camera. US Patent US4958231A. Hamamatsu Photonics K.K. Retrieved from https://patents.google.com/patent/US4958231A/en
Industry Reports
- Global Streak Camera Market Analysis 2023 by Verified Market Reports. This report offers a comprehensive overview of the current market landscape for streak cameras, including trends, challenges, and growth opportunities.
- Market Insightful Edge. (2024, February 8). High speed cameras market size projections: Navigating future trends and growth scope until 2031. LinkedIn. Retrieved from https://www.linkedin.com/pulse/high-speed-cameras-market-size-projections-navigating-qdbbf/
We believe these documents are essential for those who wish to delve deeper into the technical specifics or the historical and future contexts of streak camera technology.