Terahertz imaging sits at the intersection of physics, materials science, and engineering, leveraging electromagnetic waves in the terahertz range to penetrate surfaces, identify molecular structures, and reveal data invisible to conventional imaging techniques. With wavelengths capable of detecting non-metallic objects and differentiating between chemical compounds, terahertz imaging is advancing fields from medical diagnostics to industrial quality control and security. This guide delves into the science of THz technology, examining its unique properties, imaging techniques, and cutting-edge applications that are setting new standards in non-invasive inspection and analysis.
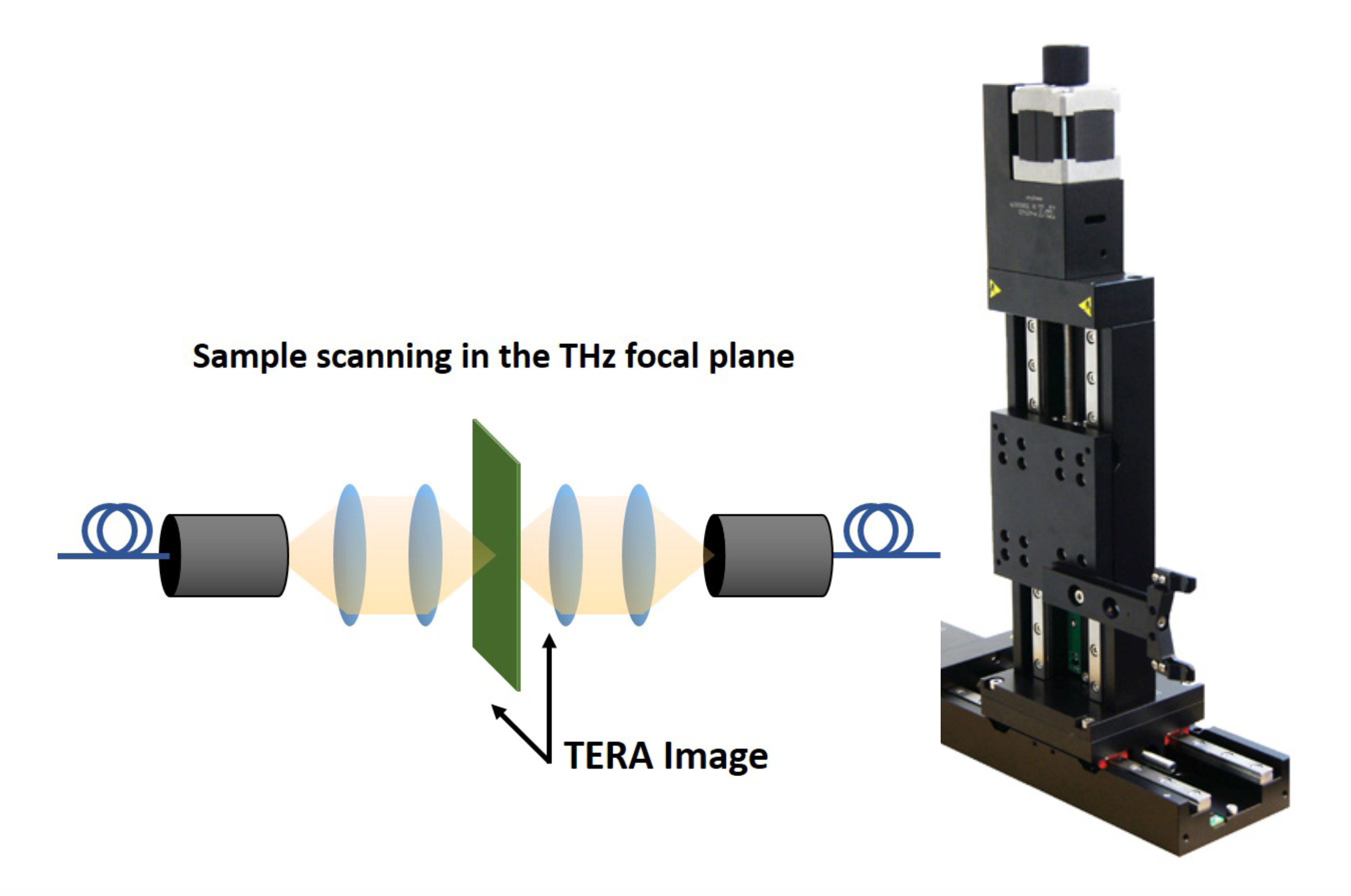
Terahertz Imaging harnesses non-ionizing terahertz waves to penetrate materials, enabling detailed inspection of hidden structures and molecular compositions. With applications in security screening, medical diagnostics, and industrial quality control, this technology is transforming non-destructive analysis across multiple fields. Image courtesy of Menlo Systems, GmbH.
Introduction
The terahertz (THz) range occupies a unique position in the electromagnetic spectrum, spanning frequencies between 0.1 and 10 THz, corresponding to wavelengths of 3 mm to 30 µm. This situates it between microwaves and infrared light, granting it properties of both. THz waves are non-ionizing, making them inherently safe for biological tissues and materials. Despite their relatively recent emergence as a practical tool, advances in generation and detection have propelled terahertz waves into the spotlight of scientific and industrial research.
Why is Terahertz Imaging Important? THz imaging has drawn significant attention due to its ability to penetrate a wide range of materials, including plastics, fabrics, ceramics, and even biological tissues, while remaining non-invasive and non-destructive. Additionally, terahertz waves provide unique spectroscopic information, as they interact with molecular vibrations and rotational states. This capability allows for chemical fingerprinting and material characterization, making THz imaging invaluable in applications requiring precision and safety.
Terahertz imaging is already proving its value in several fields. In security, it is used in airport scanners to detect concealed weapons and contraband, penetrating clothing and packaging without ionizing radiation. In medicine, studies have shown its effectiveness in identifying early-stage breast cancer and mapping burn injuries with high precision. The technology is also utilized in industrial quality control, such as inspecting tablet coatings in pharmaceuticals to ensure uniformity and detecting structural defects in ceramics and plastics. Additionally, cultural heritage researchers employ THz imaging to examine layered paintings and historical artifacts non-destructively. These real-world applications highlight its growing impact across diverse sectors.
This post is sponsored by DataRay - World Leader in Laser Beam Profiling solutions. If you are in the market, their WinCamD-QD – Quantum Dot SWIR Beam Profiler.
1. Understanding Terahertz Waves
Terahertz waves, situated between microwaves and infrared light, exhibit unique physical characteristics that enable their application in imaging and spectroscopy. Their non-ionizing nature makes them safe for biological and material investigations, while their ability to penetrate dielectric materials and interact with molecular vibrations opens new frontiers in non-destructive analysis. A detailed understanding of their properties, generation mechanisms, and detection techniques is critical for advancing terahertz-based technologies.
1.1. Basic Properties of THz Waves
Terahertz waves possess a relatively low photon energy (on the order of millielectronvolts), insufficient to cause ionization, which makes them inherently safe for probing biological tissues and sensitive materials. Their penetration capabilities allow the inspection of non-conductive materials, such as polymers, textiles, and ceramics, revealing internal structures inaccessible to optical or X-ray systems. Furthermore, their interaction with molecular vibrations and rotational transitions enables spectroscopic identification of chemical compositions, providing a unique analytical dimension compared to higher-energy radiation.
1.2. Generation and Detection of THz Waves
Advances in terahertz generation and detection have driven its adoption in scientific and industrial settings.
- THz Generation:
- Photoconductive Antennas (PCAs): Widely used in time-domain spectroscopy (TDS), PCAs generate broadband terahertz pulses when excited by femtosecond laser pulses.
- Quantum Cascade Lasers (QCLs): Emit continuous-wave terahertz radiation through intersubband transitions in semiconductor superlattices, offering high spectral purity.
- Nonlinear Optical Methods: Techniques like optical rectification and difference-frequency generation exploit nonlinear crystals to produce terahertz radiation from ultrafast lasers.
- THz Detection:
- Electro-Optic Sampling: A precise method where the interaction of terahertz fields with electro-optic crystals induces changes in birefringence, enabling time-domain measurements.
- Bolometers: Highly sensitive thermal detectors capable of measuring weak terahertz radiation by detecting temperature changes.
- Photoconductive Antennas: Dual-purpose devices that can detect terahertz waves in TDS setups with high temporal resolution.
The synergy between broadband and continuous-wave sources with high-sensitivity detectors has facilitated terahertz imaging’s rapid development, enabling its integration into fields ranging from material characterization to biomedical diagnostics.
2. Components of a Terahertz Imaging System
A terahertz imaging system integrates specialized components to generate, manipulate, and analyze terahertz radiation, with sources, detectors, optical elements, and data processing units forming the core of these setups.
2.1. THz Sources
Terahertz sources are categorized into broadband time-domain sources and narrowband continuous-wave (CW) sources. Time-domain systems typically employ ultrafast lasers in conjunction with photoconductive antennas or nonlinear crystals, producing broadband pulses suitable for spectroscopic applications and material characterization. CW sources, such as quantum cascade lasers (QCLs) and frequency-multiplied microwave oscillators, deliver stable and tunable radiation for high-resolution imaging. Broadband sources provide a wide spectral range, essential for analyzing complex materials, while CW sources are preferred for precision imaging tasks due to their narrow linewidth and high spectral purity.
2.2. THz Detectors
Detectors in terahertz systems are designed to convert terahertz radiation into measurable signals, with the choice of detector tailored to the application. Photoconductive antennas are highly efficient for time-domain systems, offering simultaneous temporal and spectral resolution. Thermal detectors, such as bolometers, excel in detecting low-power CW radiation but are limited by their slower response times. Electro-optic sampling is another widely used technique, utilizing the birefringence induced in crystals by terahertz fields for precise field measurements. Advances in room-temperature detection technologies, including pyroelectric sensors and emerging graphene-based devices, have broadened the range of applications for terahertz imaging systems.
2.3. Optics and Focusing Elements
The manipulation of terahertz waves requires specialized optics to minimize losses and maintain beam quality. Common components include high-resistivity silicon lenses, Teflon optics, and parabolic mirrors. Parabolic mirrors, in particular, are used to focus terahertz beams with minimal aberration, critical for high-resolution imaging systems. Waveguides and photonic crystals are also employed to direct terahertz waves with high precision, while emerging metamaterials offer potential for advanced beam shaping and compact optical designs.
2.4. Data Acquisition and Processing
Data acquisition in terahertz imaging relies on capturing terahertz signals and converting them into interpretable formats, such as spectral or spatial data. In time-domain systems, the electric field of the terahertz pulse is measured as a function of time, requiring precise synchronization between the source and detector. Fourier Transform techniques are used to convert time-domain data into frequency-domain spectra, providing detailed information about material properties. In imaging applications, algorithms for tomographic reconstruction and machine learning techniques are increasingly employed to enhance spatial resolution and image quality. These methods enable the extraction of complex structural and compositional information from terahertz measurements, supporting advanced applications in various fields.
3. Types of Terahertz Imaging Techniques
Terahertz imaging techniques are categorized based on how the terahertz waves interact with the target material. The primary modes are transmission imaging, reflection imaging, and spectroscopic imaging, each with distinct mechanisms and applications.
3.1. Transmission Imaging
Transmission imaging involves directing terahertz waves through a material and measuring the transmitted radiation on the opposite side. The attenuation and phase shift of the terahertz waves provide information about the internal structure and composition of the material. This technique is particularly useful for analyzing non-metallic, non-conductive objects and is widely employed in material characterization and quality inspection. For instance, in the pharmaceutical industry, transmission imaging is used to assess the uniformity of coatings on tablets, ensuring product consistency. In manufacturing, it helps detect voids or defects in plastics and composites. However, the technique is limited to materials that allow sufficient transmission of terahertz radiation, making it less suitable for highly opaque or metallic objects.
3.2. Reflection Imaging
Reflection imaging measures the terahertz waves reflected from the surface or internal interfaces of a material. This technique is ideal for security applications, such as detecting concealed weapons or contraband under clothing, and for medical diagnostics, where it is used to analyze skin conditions and burns. Reflection imaging is particularly effective for opaque materials or situations where only one side of the object is accessible. For example, in skin cancer detection, reflected terahertz waves provide high-contrast images of cancerous versus healthy tissue, leveraging differences in water content. The method’s sensitivity to surface and subsurface features makes it a powerful tool for applications requiring precise spatial resolution.
3.3. Spectroscopic THz Imaging
Spectroscopic imaging combines terahertz imaging with spectroscopic analysis to reveal material composition and molecular signatures. By analyzing the frequency-dependent absorption and refractive index of terahertz waves, this technique identifies molecular structures and distinguishes between different substances. Spectroscopic THz imaging is used extensively in chemical analysis and material identification. For example, it can differentiate between polymorphs in pharmaceutical compounds or detect explosives and narcotics in security screening. Additionally, in cultural heritage preservation, spectroscopic imaging is employed to study the chemical composition of pigments and binders in historical artwork without causing damage. Its ability to provide both spatial and spectroscopic data makes it invaluable for applications requiring detailed molecular insight.
Each of these techniques addresses specific imaging requirements, with their selection driven by the material properties, desired resolution, and application context.
4. Key Applications and Case Studies
Terahertz imaging has found practical applications across a range of fields due to its unique ability to penetrate materials, provide non-destructive analysis, and identify chemical compositions. Below are some of its key applications with real-world case studies.
4.1. Security and Screening
Terahertz imaging is widely used in security to detect concealed weapons, explosives, and other illicit substances. Unlike X-ray systems, THz imaging can penetrate clothing and non-metallic packaging while remaining non-ionizing and safe for humans. A notable example is its deployment in airport security scanners, where terahertz systems enable the identification of concealed threats with high accuracy and minimal privacy concerns by using non-intrusive imaging methods. Additionally, its ability to differentiate chemical signatures is being explored to enhance detection of explosives and narcotics in mail and cargo screening.
4.2. Medical Imaging
In medical diagnostics, terahertz imaging shows promise for early detection of skin cancers, such as melanoma, by exploiting differences in water content between healthy and cancerous tissues. Studies have demonstrated the ability of THz imaging to map burn injuries, providing detailed insight into burn depth and severity, aiding in treatment planning. Unlike X-rays and MRI, THz imaging is non-ionizing and provides high-contrast images of soft tissues without requiring contrast agents. Research continues to expand its use in fields such as dentistry and ophthalmology, offering a safer and more detailed alternative to traditional imaging techniques.
4.3. Industrial Quality Control
Terahertz imaging is a powerful tool for non-destructive testing in industrial settings. It is used in the pharmaceutical industry to inspect tablet coatings and detect defects, ensuring uniformity and quality compliance. In electronics, THz imaging helps in identifying structural defects and misalignments in circuit boards and semiconductors without damaging components. For example, manufacturers of multilayered plastic composites use terahertz systems to detect voids or delamination that might compromise product integrity. These applications highlight its role in improving manufacturing standards and minimizing waste.
4.4. Art and Cultural Heritage Conservation
THz imaging is increasingly being used in the analysis and preservation of artwork and historical artifacts. It can penetrate multiple layers of paint or varnish, revealing hidden sketches, previous restorations, or underlying structural details without harming the artwork. For instance, museums have employed terahertz systems to study the layering and composition of Renaissance paintings, providing insights into the techniques and materials used by historical artists. In archaeological research, THz imaging has been applied to analyze fragile manuscripts and historical objects, uncovering details that remain invisible to traditional imaging methods.
5. Challenges and Limitations of Terahertz Imaging
Despite its potential, terahertz imaging faces several challenges that hinder its broader adoption. One of the primary technical limitations is the low power output of terahertz sources, which restricts the imaging range and signal-to-noise ratio, especially for thick or highly absorptive materials. Additionally, terahertz imaging systems often struggle with resolution constraints due to the relatively long wavelengths compared to optical systems, limiting the level of detail in captured images.
Environmental factors further complicate its application, as humidity and atmospheric absorption significantly attenuate terahertz waves over longer distances. This makes outdoor or open-air applications difficult without specialized environmental controls. Moreover, the cost of terahertz components, including sources, detectors, and optics, remains prohibitively high for many industries.
The complexity of system design, requiring precise synchronization and alignment of components, adds to the challenge, often necessitating expertise in physics, engineering, and signal processing. These limitations currently confine terahertz imaging to niche applications where its unique capabilities outweigh its challenges, though ongoing advancements in technology aim to address these barriers.
6. Future Directions in Terahertz Imaging
The future of terahertz imaging is driven by advancements in source and detector technologies, along with expanding applications across diverse industries. Researchers are focusing on improving the efficiency and compactness of terahertz sources, such as quantum cascade lasers (QCLs), to provide higher power outputs and broader spectral coverage while maintaining practical sizes. Similarly, innovations in detector technologies, including room-temperature semiconductor detectors and graphene-based sensors, are enhancing sensitivity and reducing operational constraints. These improvements aim to lower costs and make terahertz imaging systems more accessible.
In data processing, researchers actively explore machine learning to streamline terahertz image analysis. They train algorithms on terahertz datasets to improve resolution, detect patterns in noisy data, and classify materials based on their unique terahertz signatures. For example, AI tools automate defect detection in industrial applications and distinguish cancerous tissues from healthy ones in medical diagnostics.
Emerging applications are also expanding the scope of terahertz imaging. In agriculture, terahertz systems are being tested for analyzing moisture content in crops and detecting contaminants in food. Autonomous vehicles may benefit from terahertz imaging for non-visual environmental sensing, particularly under adverse weather conditions where optical systems struggle.
Conclusion
Terahertz imaging represents a versatile and transformative technology, bridging gaps across industries with its unique ability to penetrate materials, provide non-destructive analysis, and identify molecular compositions. From enhancing security and medical diagnostics to improving industrial quality control and preserving cultural heritage, the potential applications of terahertz imaging are vast and continually expanding. Its non-ionizing nature and capability to deliver spectroscopic and spatial insights make it a valuable tool for addressing challenges that conventional methods cannot.
Advancements in terahertz sources, detectors, and data processing technologies actively drive the adoption of terahertz imaging by addressing current limitations. Researchers, engineers, and scientists can explore its possibilities within their fields, leveraging this technology to create innovative solutions. Terahertz imaging evolves rapidly, enhancing existing methodologies and unlocking new applications across science, industry, and other domains.