Definition and Scope of Terahertz Sources and Radiation (0.1 THz to 10 THz): Terahertz (THz) radiation, occupying the electromagnetic spectrum between microwaves and infrared light, encompasses frequencies from approximately 0.1 THz to 10 THz. This translates into wavelengths ranging from 30 μm to 3 mm. Terahertz waves, often referred to as the “terahertz gap,” have been a subject of intense research due to their unique position in the electromagnetic spectrum, offering a blend of properties from both sides – the penetrative power of microwave radiation and the high resolution of infrared light. This distinctive range makes terahertz radiation a key player in various advanced technological applications.
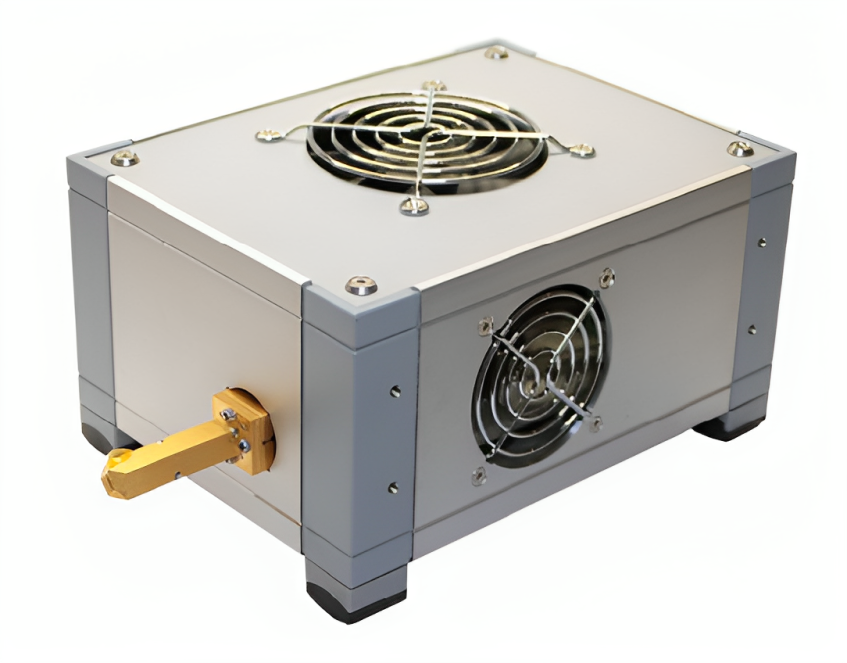
High-Power Terahertz Wave Source by Terasense Group – The Forefront of Terahertz Technology Innovation. As seen on FindLight.
Overview of Terahertz Technology Development, particularly since the 1990s: The journey of terahertz technology has been one marked by rapid evolution, especially since the 1990s. Initially limited by the challenges in generating and detecting these waves, advancements in photonics and electronics have led to significant breakthroughs. From quantum cascade lasers to photoconductive antennas, each development has contributed to making terahertz technology more accessible and applicable. Today, terahertz sources are not just scientific curiosities; they are vital tools in diverse fields ranging from security scanning to biomedical imaging, underscoring the importance of continued innovation in this area.
With this article our aim is to provide a comprehensive overview of the current state of terahertz sources, delving into their types, functionalities, and applications. By equipping you with in-depth technical knowledge, we aspire to aid in making informed decisions, whether for research, industrial use, or technological development. Thus, whether you are an engineer seeking detailed technical information or a prospective buyer looking to understand the nuances of terahertz sources for practical applications, this guide aims to address all your needs comprehensively.
In this article we cover:
- Fundamentals of Terahertz Radiation
- Types of Terahertz Sources and Their Mechanisms
- Performance Parameters and Specifications
- Applications of Terahertz Sources in Science and Industry
- Design Considerations and Technological Challenges
- Buyer’s and Engineer’s Guide to Selecting Terahertz Sources
- Future Trends and Evolving Technologies
- Conclusion
- References and Further Reading on Terahertz Sources
1. Fundamentals of Terahertz Radiation
Terahertz radiation represents a fascinating and somewhat elusive segment of the electromagnetic spectrum, lying between microwaves and infrared light. This spectrum is characterized by frequencies ranging from 0.1 THz to 10 THz, corresponding to wavelengths of 3 mm to 30 μm. Occupying a transitional zone, terahertz radiation inherits certain properties from both its neighboring spectra: it possesses the penetration capabilities of microwaves while retaining the higher frequency benefits of infrared, such as improved resolution in imaging. This unique positioning is pivotal, as it opens up possibilities for applications that are unfeasible with either microwaves or infrared waves alone.
Unique Properties and Challenges in Generating Terahertz Waves
The generation and manipulation of terahertz waves present unique challenges. Traditional electronic approaches, efficient at microwave frequencies, struggle to reach the higher terahertz range, while optical methods, effective in the infrared domain, often fall short in power and efficiency when scaled down to terahertz frequencies. This “terahertz gap” has necessitated innovative approaches to source development. Terahertz waves also exhibit distinct propagation characteristics; they can penetrate materials like clothing and packaging, yet are non-ionizing and relatively safe for biological tissues, making them suitable for a variety of sensitive applications.
Historical Perspective and Technological Milestones
The exploration of terahertz radiation began in earnest in the late 20th century. Initial efforts were met with limited success due to the challenges in generating and detecting these waves efficiently. However, the 1990s marked a turning point, with significant strides made in both aspects. Key milestones include the development of quantum cascade lasers tailored for terahertz frequencies, advances in photoconductive antennas for both emission and detection, and the adaptation of free-electron lasers for high-power applications. The continuous evolution of terahertz technology is marked by increasing power outputs, miniaturization of components, and a broader understanding of terahertz interactions with various materials. Today, terahertz technology is not only a vital tool in scientific research but also finds its footing in practical applications such as security, communications, and medical imaging.
2. Types of Terahertz Sources and Their Mechanisms
Terahertz radiation has become increasingly pivotal in numerous applications, from spectroscopy to imaging. However, generating and harnessing this radiation presents a unique set of challenges, chiefly due to its position in the spectrum. Over the years, a diverse array of sources has been developed to produce terahertz waves, each with its own set of advantages and constraints. This section delves into the various types of terahertz sources, shedding light on their underlying mechanisms, and offering a comparative insight into their efficiencies and applications.
Sources Derived from Microwave Technology: High-Frequency Oscillators (Gunn Diodes, Impatt Diodes, Resonant Tunneling Diodes): Microwave-derived terahertz sources primarily include high-frequency oscillators like Gunn, Impatt, and resonant tunneling diodes. These devices, optimized for emitting at the lower end of the terahertz spectrum, face significant challenges in scaling to higher frequencies. Although capable of reaching terahertz frequencies, their output power and efficiency drastically reduce as frequency increases. One of the primary limitations of microwave technology in terahertz applications is the steep decline in output power and efficiency at higher frequencies. This is a significant drawback, making these sources less practical for applications requiring substantial power outputs at high terahertz frequencies.
Harmonic Generation in Nonlinear Electronic Devices: Harmonic generation involves creating higher frequency waves from a lower frequency source, using nonlinear electronic devices. Although this technique enables reaching higher frequencies, it typically results in lower output powers due to the constraints in power conversion efficiency in these devices.
Free-Electron Lasers and Synchrotrons: Free-electron lasers and synchrotrons are capable of emitting very high powers in the terahertz range. However, their massive size and prohibitive cost significantly limit their practicality for widespread commercial or industrial applications, confining their use primarily to research environments.
Gas Lasers: Molecular Gas Lasers (e.g., CO2-pumped Methanol Lasers): Molecular gas lasers, such as CO2-pumped methanol lasers, exploit molecular transitions to produce discrete frequencies within the terahertz range. These gas lasers typically deliver output powers ranging from a few milliwatts to tens of milliwatts. Their discrete frequency generation capability is significant for specific applications requiring precise control over the emitted frequency.
Quantum Cascade Lasers: Quantum cascade lasers (QCLs) have been specifically developed and optimized for terahertz emission. These semiconductor lasers can be tuned within a limited frequency range and are notable for their compact size, although they require cryogenic cooling systems to operate efficiently.
Photoconductive Antennas: Photoconductive antennas serve dual purposes in terahertz technology – both as generators and detectors of terahertz radiation. They typically consist of a semiconductor material equipped with metallic stripes, functioning under the principles of optical sampling technology. These antennas can operate in either continuous-wave or pulsed modes. In the continuous-wave mode, particularly, they are known as terahertz photomixers, capable of generating highly pure and tunable terahertz radiation.
Parametric Interactions in Nonlinear Crystals: Difference Frequency Generation Techniques: This method involves generating terahertz radiation through the difference frequency generation of two closely related optical waves. It represents a significant approach in terahertz source technology, leveraging nonlinear crystal materials. Optical Rectification: Optical rectification is a method used for converting optical frequencies directly into terahertz frequencies. However, this process faces challenges related to efficiency and beam divergence due to the long wavelengths of terahertz radiation.
Optical Rectification in Gases: Optical rectification can also occur in gases, leading to terahertz wave emission. This method is advantageous for generating broader bandwidths and higher pulse energies, although it requires careful phase control for high efficiency.
Emerging Technologies: Landau level lasers, a promising area in terahertz technology, are based on the use of strong magnetic fields in semiconductors to create Landau levels. While promising, this technology still requires significant development to achieve satisfactory performance levels.
Other Innovative Approaches: Apart from these mainstream methods, there are continuous efforts in exploring new materials and mechanisms to bridge the terahertz gap more efficiently. These innovations focus on improving power output, efficiency, tunability, and compactness of terahertz sources.
3. Performance Parameters and Specifications
When evaluating terahertz sources, understanding their performance parameters and specifications is crucial for both engineers and buyers. These parameters not only determine the applicability of a terahertz source for a specific use case but also influence the overall system design and feasibility. This section outlines the key performance metrics that define the capabilities and limitations of terahertz sources.
Frequency Range, Tunability, and Spectral Coverage
- Frequency Range: The frequency range of a terahertz source dictates its applicability in various fields. Sources range from 0.1 THz to 10 THz, with specific technologies favoring certain segments within this spectrum.
- Tunability: Some terahertz sources offer tunability, allowing users to select specific frequencies within a given range. This feature is vital for applications like spectroscopy, where precise frequency control is necessary.
- Spectral Coverage: The width of the spectrum covered by a source affects its versatility. Broadband sources offer wide spectral coverage, making them suitable for applications requiring a range of frequencies, whereas narrow-band sources are more focused.
Output Power Levels and Efficiency Considerations
- Output Power: The power output of terahertz sources varies widely, from milliwatts to several watts, impacting their suitability for different applications. High-power sources are necessary for applications like imaging, where penetration depth is key.
- Efficiency: Power efficiency is a critical parameter, especially for portable or battery-operated applications. Sources with high efficiency are more practical for prolonged use and are environmentally favorable.
Thermal Management and Cooling Requirements
- Cooling Needs: Certain terahertz sources, like quantum cascade lasers, require cryogenic cooling systems, which can be a limiting factor in terms of size and portability.
- Thermal Management Solutions: Effective thermal management is vital for maintaining performance and longevity. This includes considerations for heat sinks, airflow, and in some cases, active cooling systems.
Size, Portability, and Practical Use Constraints
- Physical Dimensions: The size of a terahertz source impacts its deployability in different environments. Compact sources are advantageous for handheld or space-constrained applications.
- Portability: Factors like weight, power requirements, and cooling systems influence the portability of a terahertz source. Portable devices are essential for field applications and remote sensing.
- Practical Use Constraints: This includes considerations like ease of integration with other systems, maintenance requirements, and operational durability under varying environmental conditions.
A thorough understanding of these performance parameters is essential for selecting the right terahertz source for specific applications. It will also ensure that the chosen technology aligns with the intended use, environmental constraints, and performance expectations.
4. Applications of Terahertz Sources in Science and Industry
Terahertz technology, owing to its unique place in the electromagnetic spectrum, opens up a plethora of applications in both scientific research and industrial domains. The distinct properties of terahertz radiation, such as its ability to penetrate various materials without the ionizing effects of X-rays, make it an invaluable tool in numerous fields. This section explores the diverse applications of terahertz sources, highlighting their roles in spectroscopy, communications, imaging, and their varying implications in research versus industrial settings.
Terahertz Spectroscopy
- Material Analysis: Terahertz spectroscopy is pivotal in material science for studying chemical compositions and physical properties. It offers non-destructive analysis, crucial for examining sensitive or precious materials.
- Pharmaceutical Applications: In the pharmaceutical industry, terahertz spectroscopy is employed for quality control, especially in monitoring coating processes and detecting impurities in drugs.
- Biological Research: It’s also used in biological research to study the properties of biomolecules, tissues, and fluids, owing to its non-ionizing nature and sensitivity to water content.
Communications
- High-Speed Wireless Communications: The high frequency of terahertz waves allows for ultra-fast data transmission, opening pathways for next-generation wireless communication technologies.
- Secure Communications: Due to the directional nature and limited range of terahertz waves, they offer potential for secure communication channels in military and strategic applications.
Terahertz Imaging
- Security Screening: Terahertz imaging is extensively used in security checkpoints for detecting concealed weapons and explosives, as these waves can penetrate clothing and non-conductive materials.
- Medical Diagnostics: In the medical field, terahertz imaging offers a safer alternative to X-rays for certain diagnostic applications, including skin and breast cancer screening.
Research Applications versus Industrial and Commercial Uses
- Research Tools: In academic and research institutions, terahertz sources are critical in advancing knowledge in physics, material science, and engineering. They are instrumental in exploratory research and experimental setups.
- Industrial and Commercial Adaptation: In industry, the focus is on practical applications like quality control in manufacturing, non-destructive testing, and process monitoring. The scalability, robustness, and cost-effectiveness of terahertz technology are key considerations in these settings.
- Evolving Uses: The applications of terahertz technology are continuously evolving, with new uses emerging in sectors like agriculture for moisture detection, in environmental monitoring, and even in art conservation.
In summary, terahertz sources are versatile tools that bridge the gap between scientific exploration and practical industrial applications. As technology advances, their role in both domains is set to expand, unlocking new possibilities and solutions.
5. Design Considerations and Technological Challenges
The design and development of terahertz sources pose a unique set of challenges, largely stemming from the specific physical properties of terahertz radiation and its interaction with various materials and components. Key among these challenges are issues related to noise, signal-to-noise ratio (SNR), beam quality, integration with system components, environmental sensitivity, and overall durability of the device.
Noise and Signal-to-Noise Ratio: One of the primary concerns in terahertz source design is managing noise levels to achieve an optimal signal-to-noise ratio. Given that many terahertz applications, such as imaging and spectroscopy, depend on the detection of subtle differences in signal strength, high levels of inherent system noise can significantly degrade performance. Designers often need to balance between maximizing output power and minimizing electronic and thermal noise, which becomes increasingly challenging at higher frequencies. Innovative techniques in electronic design, component selection, and signal processing are continuously developed to enhance SNR in terahertz systems.
Beam Quality: The quality of the terahertz beam, characterized by its coherence, phase stability, and divergence, is another critical aspect. Poor beam quality can limit the effectiveness of terahertz sources, particularly in applications requiring precise focus or long-distance transmission. Advanced optical design, precise alignment of components, and the use of high-quality materials are essential to maintain the integrity of the terahertz beam.
Integration with System Components: Integrating terahertz sources into larger systems, whether for scientific instrumentation or industrial applications, presents its own set of challenges. Compatibility with other system components, such as detectors, modulators, and data processing units, is crucial. This often requires a multidisciplinary approach in design, encompassing expertise in photonics, electronics, material science, and computer engineering. Additionally, the miniaturization of terahertz sources for portable or handheld devices further complicates integration, necessitating innovations in compact and efficient design.
Environmental Sensitivity and Durability: Terahertz sources are often sensitive to environmental conditions such as temperature, humidity, and atmospheric pressure. These factors can affect both the performance and longevity of the device. For instance, some terahertz sources, like quantum cascade lasers, require cryogenic cooling, which adds complexity to their operation and limits their practicality in certain environments. Ensuring durability and consistent performance across a range of environmental conditions is key, especially for commercial and industrial applications where sources may be subjected to harsh or variable conditions.
In conclusion, designing and developing terahertz sources demands a comprehensive understanding of both the underlying physics and the practical application challenges. Continuous advancements in materials science, electronics, and photonics are critical to overcoming these challenges, paving the way for more efficient, robust, and versatile terahertz sources.
6. Buyer’s and Engineer’s Guide to Selecting Terahertz Sources
When selecting a terahertz source, buyers and engineers face a multitude of factors to consider. This guide is designed to assist in navigating through these considerations, ensuring that the chosen terahertz source aligns with specific application needs, cost constraints, operational longevity, and vendor reliability.
Matching Source Types to Specific Applications: The first step is to identify the most suitable type of terahertz source for the intended application. Different applications have varying requirements in terms of frequency range, power output, beam quality, and tunability. For instance, applications in high-resolution imaging or non-destructive testing might benefit from sources with high power and broad spectral coverage, while applications in spectroscopy might prioritize sources with narrow linewidths and high frequency stability. Understanding the nuances of each source type, from quantum cascade lasers to photoconductive antennas, and their alignment with the application’s demands is crucial.
Evaluating Cost-Performance Trade-offs: Budget constraints often play a significant role in the selection process. High-power free-electron lasers, while powerful, might be prohibitively expensive for some labs or industries. Conversely, more affordable sources like Gunn diodes may not offer the required performance for certain advanced applications. Evaluating the cost against performance metrics like output power, frequency stability, and operational lifespan is essential. This evaluation should also consider the costs associated with ancillary equipment, like cooling systems for quantum cascade lasers.
Considerations for Long-Term Operation and Maintenance: The long-term operational and maintenance aspects are pivotal. Some terahertz sources may require frequent calibration, specialized cooling systems, or regular maintenance that could incur additional costs or downtime. Buyers should assess the total cost of ownership, including these long-term operational factors, to ensure a sustainable investment.
Vendor Evaluation: Reputation, Support, and Compliance: Finally, evaluating the vendor is as important as assessing the terahertz source itself. A vendor with a strong reputation in the terahertz community, positive customer feedback, and robust support services can be invaluable, especially for complex and high-tech devices like terahertz sources. It’s also important to consider the vendor’s compliance with industry standards and certifications, which can be critical for certain regulated industries.
In conclusion, selecting the right terahertz source involves a comprehensive evaluation of application-specific requirements, cost-performance balance, long-term operational considerations, and vendor reliability. A well-informed decision not only ensures optimal performance for the intended application but also secures a reliable and sustainable investment in the long run.
7. Future Trends and Evolving Technologies
The field of terahertz technology is rapidly advancing, with several key trends and potential breakthroughs on the horizon. These developments are expected to shape the future of terahertz sources, expanding their capabilities and applications.
Miniaturization and Power Efficiency Advances: One of the most significant trends in terahertz technology is the ongoing miniaturization of sources and improvements in power efficiency. As semiconductor technologies evolve, there is a continual push towards creating smaller, more efficient terahertz sources. This trend is not only making terahertz systems more portable and accessible but also opening up new applications in areas where space and power efficiency are critical, such as in handheld devices for security screening or in-field diagnostics. Improved power efficiency also means longer operational lifetimes and reduced operational costs, making terahertz technology more viable for a wider range of applications.
Expanding Application Horizons: Terahertz technology is increasingly finding applications beyond traditional areas such as spectroscopy and imaging. The expanding horizons include fields like wireless communications, where terahertz frequencies could enable ultra-high-speed data transfer, and in the medical sector, for non-invasive diagnostic tools. As the technology matures, we can expect to see terahertz sources being integrated into a broader array of industrial and consumer applications, driven by advancements in source power, reliability, and compactness.
Anticipated Technological Breakthroughs and Research Directions: The future of terahertz technology also holds promise for significant breakthroughs. Key areas of research include the development of room-temperature quantum cascade lasers, which could revolutionize the use of terahertz sources by eliminating the need for complex cooling systems. Advances in non-linear materials and photonic structures might lead to more efficient and tunable sources, broadening the functional spectrum of terahertz radiation. Additionally, research into hybrid technologies that combine different source mechanisms could yield sources with unprecedented flexibility and performance.
The field of terahertz technology is on the cusp of significant transformations, with advances in miniaturization, power efficiency, and breakthroughs in materials and laser technology. These developments are not only enhancing the capabilities of terahertz sources but are also paving the way for their integration into a diverse array of new and existing applications, marking an exciting era for terahertz technology.
8. Conclusion
Recap of Key Insights: This article has comprehensively explored terahertz sources, highlighting their development from microwave technology to advanced quantum cascade lasers and photoconductive antennas. Terahertz radiation, with its unique position in the electromagnetic spectrum, presents specific challenges and opportunities, especially in fields like spectroscopy, imaging, and communication. We’ve discussed critical performance parameters such as frequency range, output power, and efficiency, emphasizing the balance between technical capabilities and practical limitations. The integration of these sources in various applications underscores the ongoing need to address design considerations and technological challenges.
The Forward Path in Terahertz Source Technology: The future of terahertz technology offers ongoing miniaturization, improved power efficiency, and broader application horizons. Advances in this field are expected to lead to more compact, potent, and energy-efficient sources, potentially revolutionizing areas like high-speed communications and non-invasive diagnostics.
Research in terahertz technology is rapidly evolving, suggesting imminent breakthroughs such as room-temperature quantum cascade lasers and more efficient, tunable source mechanisms. The integration of different source methods for enhanced performance is also a promising direction.
In summary, terahertz source technology is at a crucial point of growth, with its potential applications just beginning to unfold. The field’s advancements will make terahertz sources an increasingly vital part of our scientific and technological landscape, promising transformative applications across various sectors.
9. References and Further Reading on Terahertz Sources
This article has drawn upon a diverse range of sources, including academic papers, industry reports, and patent literature, to provide a comprehensive overview of terahertz sources. We also recommended the following sources and references for those seeking a deeper understanding or more technical details:
- Academic Journals: Explore journals like “Journal of Infrared, Millimeter, and Terahertz Waves” and “IEEE Transactions on Terahertz Science and Technology” for peer-reviewed papers detailing recent advancements and research in terahertz technology.
- Patent Literature: Search patent databases for recent filings related to terahertz source technologies. Patents often give an early indication of where research and development efforts are being directed in the industry.
- Books and Textbooks: Several comprehensive texts are available that delve into the physics, engineering, and applications of terahertz technology. These are invaluable for both students and professionals.
- Conference Proceedings: International conferences such as the International Conference on Infrared, Millimeter, and Terahertz Waves (IRMMW-THz) regularly publish proceedings that contain cutting-edge research and developments in the field.
- Online Resources: Websites like the Terahertz Sources category page on FindLight offer a curated selection of products and technologies in the terahertz domain, making it a valuable resource for comparing specifications and vendors.
- Technical Manuals and Product Literature: For practical, hands-on insight, technical manuals and product datasheets from terahertz source manufacturers provide detailed specifications and operational guidelines.
These resources collectively offer a thorough understanding of the current state and future potential of terahertz sources, guiding both technical professionals and prospective buyers in navigating this dynamic field.