Introduction
In this article we will discuss the evolution of mid-infrared beam delivery methods. Since their development in the 1950s, optical fibers have been fundamental to the world of modern communication and information exchange. While the concept of guiding light through a transparent medium has roots dating back centuries, the first successful and lossless demonstration of light traveling through optical fibers dates to the mid-20th century. With their ability to transport vast amounts of data at the speed of light over long distances and with very low loss, optical fibers have become the backbone of all communication networks we use in our day-to-day lives. Nevertheless, the utility of optical fibers extends beyond the realm of telecommunications, as their integration has spilled into an array of diverse fields, including the medical sector and laser welding, where precision and adaptable beam delivery are prerequisites for success.
This article is sponsored by art photonics GmbH, a leading developer and manufacturer of fibers and fiber optic components and assemblies used in medial, laser, academic and other fields
Properties of Fused Silica Fibers
Among the various types of optical fibers, fused silica fibers stand out as one of the most widely used variants. Composed primarily of silicon dioxide glass, these fibers excel at propagating wavelengths across a broad spectrum, spanning from the deep ultraviolet at 180 nm to the near-infrared reaching 2 μm. However, their performance begins to degrade when confronted with longer wavelengths. For wavelengths exceeding 2 μm, fused silica fibers exhibit substantial absorption, effectively diminishing the power that traverses the fiber. This absorption effect becomes even more pronounced over extended propagation distances. Therefore, to preserve the integrity of the data carried by the beam, interventions such as signal amplification and recovery methods become necessary.
To overcome the limitations of fused silica fibers in longer wavelength regions, the emergence of mid-infrared (MIR) optical fibers has been instrumental. These specialized fibers are designed to operate effectively in the mid-infrared range, and they offer distinct advantages when extended fiber lengths are required.
3D model showing the structure of an optical fiber. Inner Core or Core where 90% of the light propagates and Outer Cladding. The third layer is a Plastic Sheath which serves as a protective layer. Courtesy of Goalfinder.
History of Infrared Materials and the Surge of Infrared Optical Fibers
During the 1960s, research into infrared materials surged and attracted considerable attention. This surge was fueled by the growing demand for thermal sensors, which could only be realized through the utilization of infrared materials. Additionally, the fervor of the space race contributed significantly to the heightened interest in these materials. Among the materials that emerged are Germanium, boasting a spectral range spanning from 2 μm to 18 μm, calcium fluoride, which covers regions in the deep ultraviolet to the far-infrared at 12 μm, and chalcogenide glasses with varying spectral ranges depending on their composition.
These materials were later exploited in the fabrication of mid-IR optical fibers.
For instance, fluoride fibers are known for possessing the lowest optical losses in the 1.5-3 um range. This makes them ideal hosts for delivering high-power lasers,. They are also characterized by a high temperature stability that is maintained for temperatures as high as 250 ͦ C. Additionally, they are non-toxic and do not pose any health concerns to the user. Saphire fibers are another example of non-toxic optical fibers characterized by high transmission and low loss properties. They are also employed in the delivery of high-power lasers and in the fabrication of spectroscopy and surgery probes. However, it is important to note that infrared glass fibers are limited by their narrow transmission windows. This restricts their applicability to specific domains where a wider spectral range is more desired.
Types of Mid-Infrared Optical Fibers
CIR Optical Fibers
To expand the transmission windows of infrared fibers, researchers spent many years investigating potential compositions of infrared materials and fabrication techniques. Those efforts led to the advent of what are considered some of the best optical fibers, chalcogenide infrared fiber (CIR). These fibers are step-index optical fibers comprised of two main parts: a core and a cladding. They can propagate wavelengths of light from 1.5 um up to 6 um with very low attenuation.
These fibers are meticulously crafted through a multi-step manufacturing process. It begins with the formulation of chalcogenide glass by blending chalcogen elements like sulfur, selenium, and tellurium with other elements, often metals, to achieve the desired optical and mechanical properties. The glass composition is then heated and drawn into a thin fiber form through a process called fiber drawing. To enhance the mechanical strength of these brittle structures, protective coatings are applied, and the ends are polished.
CIR fibers can propagate light for a distance of up to 50 m with little loss. Their unique properties make them invaluable in various fields. In spectroscopy, CIR fibers enable precise identification and quantification of gases and chemical compounds, making them pivotal in environmental monitoring, gas analysis, and chemical process control. In the realm of medical imaging and diagnostics, they find utility in mid-infrared spectroscopy, thermal imaging, and non-invasive tissue analysis, offering insights into diseases and biological processes. The defense and security sector harnesses CIR fibers for infrared sensors and military applications benefiting from their ability to transmit mid-infrared light for accurate threat detection.
Additionally, CIR fibers are essential in industrial settings for process control, particularly in petrochemical industries, where they enable real-time monitoring of processes and quality control. Their flexibility and bendability facilitate their integration into complex optical systems, expanding their reach in diverse applications.
PIR Optical Fibers
Another type of IR fiber that spans a wider transmission range is polycrystalline or PIR fibers. They are designed to support wavelengths between 3 um and 18 um, particularly wavelengths in the fingerprint region. The fingerprint region spans roughly from 2.5 to 15 micrometers (μm) and contains unique absorption bands associated with molecular vibrations. The fingerprint region spans roughly from 2.5 to 15 micrometers (μm) and contains unique absorption bands associated with molecular vibrations.
One of the primary applications of PIR fibers is in spectroscopy, especially in the analysis of chemical compounds and materials. The fingerprint region contains distinctive absorption lines that can be used for qualitative and quantitative analysis, enabling the identification of complex molecules and substances. Researchers and laboratories use PIR fibers in Fourier-transform infrared (FTIR) spectroscopy systems for precise molecular characterization.
Another critical application of PIR fibers is in remote sensing and environmental monitoring. PIR fibers are employed in sensors and imaging systems that detect and analyze trace gases, pollutants, and atmospheric conditions. These fibers are particularly well-suited for detecting greenhouse gases, monitoring air quality, and studying atmospheric composition.
Furthermore, PIR fibers find utility in medical diagnostics and research. They enable non-invasive analysis of biological tissues and fluids by exploiting the unique spectral signatures of various biomolecules. This facilitates applications such as disease detection, tissue characterization, and drug development.
PIR fibers are made of a silver halide material known for being non-toxic and non-hygroscopic. This material is extruded by plastic deformation under high pressure and elevated temperature to produce PIR fibers.
While PIR fibers are traditionally multimode fibers, there have been demonstration of a special technology for manufacturing single-mode fibers. The goal is to implement single-mode PIR fibers in the ESA Darwin mission program where they will serve as wavefront filters.
Design Considerations of PIR and CIR Fibers
A common challenge that is present in PIR and CIR fibers is the high Fresnel reflection at the ends of a fiber link. Due to their large index of refraction, there is a high contrast in the refractive index as light goes from propagating in air to propagating within the material. This results in reflection losses exceeding 30%. While applying anti-reflection coatings to the fiber end facets can significantly reduce those losses, their complete elimination is not guaranteed. An alternative to that would be to use another class of infrared fibers known as hollow waveguides or HWGS. As their name suggests, hollow waveguides do not have a solid core that light can propagate through. Light in these waveguides will travel through a channel or a bore created in a material.
Hollow Waveguides
Similar to CIR and PIR optical fibers, the technology behind hollow waveguides is rooted in the principle of total internal reflection, where light is confined within the hollow core due to the reflective properties of the core-to-cladding interface. This design allows for efficient light transmission while avoiding the material dispersion and absorption often encountered in solid-core fibers. The dimensions and geometries of the hollow core can be precisely engineered to manipulate the polarization and confinement of light. Hollow waveguides can be made from a range of materials, including glass, metals, and polymers, with each type tailored to specific applications.
These waveguides have found important applications in several fields. In the realm of spectroscopy, hollow waveguides are employed for the analysis of gases and volatile compounds, offering high sensitivity and rapid response times. In the medical sector, they are used in diagnostic devices for breath analysis and medical imaging techniques like mid-infrared spectroscopy. Hollow waveguides also play a critical role in laser systems, enabling the delivery of high-power laser beams and facilitating laser surgery and material processing. Their versatility, low cost, and precise control over light make hollow waveguides a valuable tool in photonics and optics.
Design Considerations of HWGs
However, hollow waveguides are more sensitive to bending than their solid-core counterparts. The TIR condition is broken in the region over which bending is applied. This leads to the light coupling out of the waveguide and being permanently lost. Depending on the radius of curvature, the transmitted power can drop by 30% or more which results in a reduction in the efficiency of the beam delivery method. This is particularly critical to applications where precision and stability are needed. Additionally, HWGs start exhibiting high losses when the numerical aperture of the incoming cone of light exceeds 0.1. In other words, these devices are limited by their small acceptance angles which make coupling light into this waveguide challenging and inefficient.
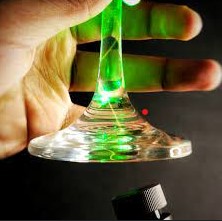
Photograph showing the total internal reflection (TIR) of a green laser beam in glass. The refractive index of glass (~1.5) is higher than that of air (~1). The high contrast in refractive indices ensures the propagation of the light through the glass. Light propagation in all optical fibers operates on the same principle. Courtesy of Wikicommons.
Summary
While CIR fibers and HWGs offer impressive capabilities, PIR fibers stand out as one of the most versatile and advantageous optical fiber options due to their extended transmission range, suitability for molecular analysis, non-toxic composition, and adaptability to single-mode applications. Not to mention, their larger NA allow them to be easily and efficiently paired with different types of sources. Their unique characteristics make them invaluable tools in fields ranging from spectroscopy and environmental monitoring to medical diagnostics and cutting-edge space missions.
Application Example of Mid-Infrared: Fiber Probes for Spectroscopy
Background on Spectroscopy
The integration of infrared (IR) fibers into probes holds paramount importance in the realm of spectroscopy analysis within the mid-infrared (MIR) spectral range, spanning from λ = 2 to 18 μm. This spectral region is particularly effective for remote in-line control of molecular composition in both industrial and laboratory processes. It owes its significance to the fact that characteristic bands of most molecular vibrations, including those of organic molecules, align with this spectral range. Consequently, IR spectra derived from mid-infrared spectroscopy offer invaluable insights into the molecular content of various substances. This analysis enables precise identification of chemical composition and facilitates real-time monitoring of changes in mixture composition.
In traditional spectroscopic approaches, a major limitation arises from the need for physical sampling followed by subsequent measurements. This hampers the realization of real-time control in various chemical processes, including polymerization and oil distillation. Furthermore, the applied control method must provide measurements immediately within the zone of the reaction, which often transpires in conditions encompassing a wide temperature range, varying pressure levels, potential mechanical vibrations, aggressive chemical components, and intense electromagnetic fields.
Fiber Probes
The solution to these challenges lies in the integration of optical fibers into probes. Optical fibers offer the capability to transmit radiation from the sample to a spectrometer while seamlessly satisfying all the aforementioned conditions. Fiber probes can be seamlessly connected with a range of spectrometers. This includes FTIR (Fourier-transform infrared), TDL (tunable diode laser), QCL (quantum cascade laser), or IR-filter spectrometers, enabling molecular analysis of liquids and gases across an extensive temperature range from 5 K to 500 K, even in the presence of strong electromagnetic fields.
In this context, PIR fibers play a pivotal role, extending the capabilities of molecular analysis to wavelengths between 3 μm and 18 μm. The unique spectral signatures within the fingerprint region offer opportunities for qualitative and quantitative analysis, making PIR fibers indispensable in applications such as disease detection, environmental monitoring, and chemical analysis. Thus, the integration of IR fibers into probes revolutionizes the landscape of molecular composition analysis, enabling real-time control and monitoring across diverse conditions and applications, with PIR fibers standing out as a versatile and indispensable choice.
Conclusion
Optical fibers have evolved significantly since the 1950s, transcending their initial role as the backbone of modern communication networks. MIR optical fibers in particular, have proven their worth across various domains, from telecommunications to medical diagnostics and cutting-edge space missions. While fused silica fibers dominate the near-infrared spectrum, their limitations in the longer wavelength regions have spurred the development of specialized mid-infrared fibers.
The history of infrared materials has paved the way for the fabrication of advanced MIR optical fibers. Among these, chalcogenide infrared fibers (CIR) have emerged as exceptional tools, capable of propagating light from 1.5 μm to 6 μm with minimal attenuation. Their applications span environmental monitoring, medical diagnostics, defense, and industrial process control.
Polycrystalline fibers have further expanded the horizons of mid-infrared spectroscopy, supporting wavelengths from 3 μm to 18 μm. PIR fibers excel in qualitative and quantitative analysis, enabling disease detection, environmental monitoring, and more. Their composition, characterized by non-toxicity and extrudability, offers versatility for various applications, including single-mode advancements.
If you liked this article you might also like “Fiber Optic Probes in PAT: Enhancing Real-Time Process Analytics“.