In this article we will take you on a journey through the enigmatic world of invisible materials, leading you to our detailed exploration of the inner workings of modern meta-materials that enable cloaking applications. Here is what we cover in brief:
- Introduction
- The Science of Invisibility: An Overview
- Historical Perspectives on Invisibility
- Types of Invisible Materials
- Manufacturing Invisible Materials
- Applications of Invisible Materials
- Ethical and Social Considerations
- Future of Invisible Materials
- Conclusion
- References and Further Reading
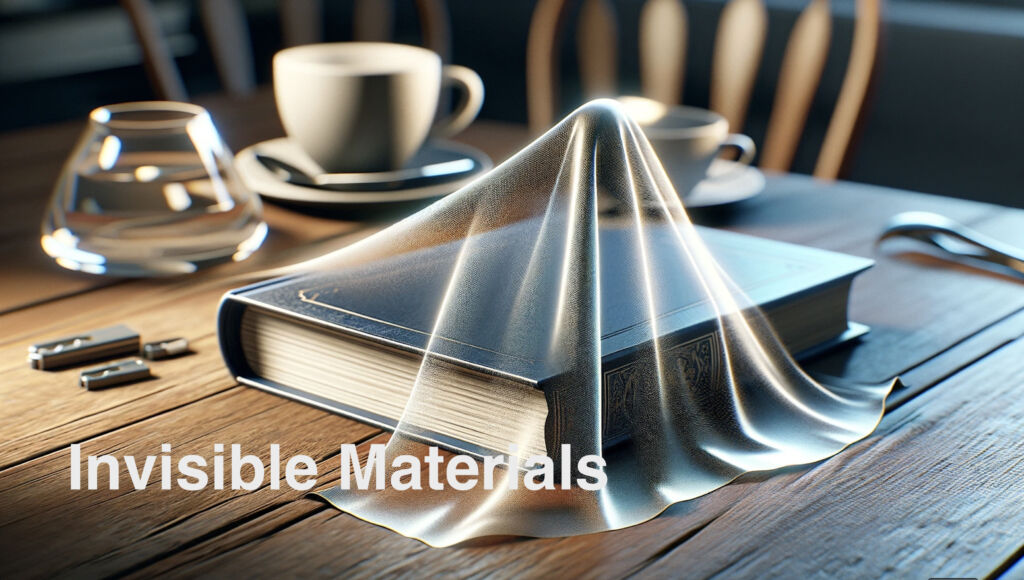
Exploring Partial Invisibility: See how an ordinary item is transformed by cloaking technology, offering a peek into a realm where visibility and invisibility intertwine. Conceptual Illustration.
1. Introduction
The pursuit of invisibility has evolved from mythical aspirations to a tangible scientific endeavor within materials science, leveraging the nuanced interplay of light and matter. This field, rooted in the principles of optics, delves into the engineering of materials with unique properties to bend, absorb, or reflect light in ways that render objects invisible or nearly so to the human eye. Such advancements are not merely academic; they have profound implications across multiple sectors, including military stealth technologies, non-invasive medical imaging, and the development of next-generation optical devices.
Central to this exploration is the concept of metamaterials, engineered composites that exhibit properties not found in naturally occurring materials, primarily their ability to manipulate electromagnetic waves. Recent studies, such as those published in Nature Photonics[1] and Advanced Materials[2], highlight the strides in metamaterials capable of steering light around objects, effectively cloaking them from view. This capability hinges on the materials’ unique refractive indices and structural compositions, which challenge traditional optical limits.
Moreover, the realm of nanotechnology introduces nanostructured materials that manipulate light at scales smaller than its wavelength, opening avenues for hyperbolic metamaterials and photonic crystals that further the cause of invisibility. The implications are vast, with potential applications ranging from rendering a military vehicle invisible to radar, to cloaking surgical tools in a medical setting for less intrusive procedures.
This article aims to unpack the layers of invisibility in materials science, from foundational theories and groundbreaking materials to the sweeping range of applications poised to redefine industry standards. Through this exploration, we illuminate the unseen, inviting engineers, optics enthusiasts, and the scientifically curious to envision a future shaped by the invisible.
2. The Science of Invisibility: An Overview
The concept of invisibility has transitioned from the realm of fantasy to a tangible scientific pursuit, facilitated by the intricate manipulation of light waves. This endeavor challenges our foundational understanding of optics and materials science, focusing on the principle of making objects unseen by effectively guiding light around them.
Manipulating Light: Refraction, Reflection, and Beyond
In the pursuit of invisibility, the interaction of light with materials plays a pivotal role. Light, an electromagnetic wave, is subject to reflection, refraction, and diffraction upon encountering various surfaces and materials. Reflection sends light waves bouncing back from surfaces, refraction bends them as they pass through different media, and diffraction causes light to spread or scatter, often leading to a mix of light and dark patterns within materials. The science of making materials invisible primarily revolves around controlling these fundamental interactions, with the objective of allowing light to traverse around an object seamlessly, as if it were not there.
Central to this science is the refractive index of materials, a measure that indicates how much a material can slow down light. Traditional optics, governed by Snell’s law, predicts the bending of light at specific angles when it passes from one medium to another, based on their refractive indices. The art of invisibility involves engineering materials with customized refractive indices to direct light precisely around objects, effectively making them ‘invisible’ by eliminating shadows and reflections.
Metamaterials: Engineering Invisibility
At the forefront of invisibility research are metamaterials, engineered composites with unique properties not found in nature. These materials are designed to possess a negative refractive index, an attribute that allows for the unconventional bending of light, opposite to what is observed with natural materials. This property is instrumental in the development of cloaking devices, as it enables the creation of materials that can bend light around objects, concealing them from view. Research highlighted in publications such as Physical Review Letters[3] illustrates the potential of metamaterials to cloak objects selectively, depending on the wavelength of light, paving the way for practical applications of invisibility.
Beyond the manipulation of light through refraction and reflection, achieving invisibility also necessitates controlling the scattering and diffraction of light. When light waves encounter an object, they tend to scatter, making the object visible. The challenge lies in designing materials that minimize this scattering effect, allowing light to pass as if the object were non-existent.
3. Historical Perspectives on Invisibility
The quest for invisibility has long captivated human imagination, intertwining myth, magic, and emerging scientific inquiry. From ancient tales of invisible cloaks found in Greek mythology to the fantastical renditions in modern literature and cinema, the idea of becoming unseen has permeated various cultures and epochs. This fascination laid the groundwork for scientific exploration, with early experiments in optics by scholars like Alhazen laying foundational principles of light manipulation.
Significant milestones in invisibility research include the development of metamaterials in the early 21st century, which marked a shift from speculative fiction to tangible scientific pursuit. These materials, capable of bending light around objects, were a leap forward in achieving optical invisibility, rooted in centuries-old theories of light and vision. Notably, the 2006 demonstration of a cloak that could divert microwaves around a copper cylinder by researchers at Duke University showcased the potential of metamaterials in rendering objects invisible, bridging the gap between lore and laboratory.
The legacy of past research continues to inspire scientists, driving innovation in materials science and optical engineering. The historical interplay between imagination and empirical study illustrates the human propensity to turn fiction into feasible technology, with the pursuit of invisibility serving as a prime example of this enduring quest.
4. Types of Invisible Materials
The advancement of invisible materials leverages sophisticated technologies to manipulate light and electromagnetic waves, making objects imperceptible to the observer. This section explores the principal materials driving this revolutionary field, including their fabrication, operational mechanisms, and real-world applications.
Metamaterials
Metamaterials are artificially engineered structures designed to exhibit properties not found in naturally occurring substances, primarily their ability to control electromagnetic waves. These materials are constructed from arrays of small, repeated units, each designed to interact with light in a specific manner. By altering the size, shape, and arrangement of these units, scientists can create materials with a negative refractive index, enabling light to bend in unnatural ways. A prominent example includes the “invisibility cloak” developed by Duke University researchers, which demonstrated the potential to guide microwaves around a copper cylinder, effectively hiding it from detection.
Nanostructured Materials
At the scale of nanometers, material properties can significantly differ from their bulk counterparts, offering unique opportunities to manipulate light. Nanostructured materials consist of tiny, structured units that can influence light waves passing through or around them. Techniques such as electron beam lithography and self-assembly are employed to create these intricate structures. An application of nanostructured materials is in the development of super-lenses that surpass the diffraction limit of conventional optics, enabling imaging of objects smaller than the wavelength of light.
Photonic Crystals
Photonic crystals are materials with periodic optical nanostructures that affect the motion of photons, similar to how the periodic potential in a semiconductor crystal affects electrons. By creating a periodic structure at scales comparable to the wavelength of light, these materials can control the flow of light within them, leading to phenomena such as photonic band gaps, which prohibit the propagation of light within a certain wavelength range. Fabrication techniques include layer-by-layer deposition and holographic lithography. Photonic crystals find applications in various fields, from enhancing the efficiency of solar cells to creating compact optical fibers that guide light with minimal loss.
Non-Hermitian Materials
A relatively novel addition to the arsenal of light-manipulating materials is non-Hermitian materials, which allow light to traverse through them without any distortion, akin to light moving through free space. These materials achieve this by locally amplifying or absorbing light, thus negating the effects of scattering and diffraction. Theoretically proposed by researchers at the Vienna University of Technology, these materials suggest a new paradigm in the quest for invisibility, emphasizing the seamless transmission of light waves through complex structures.
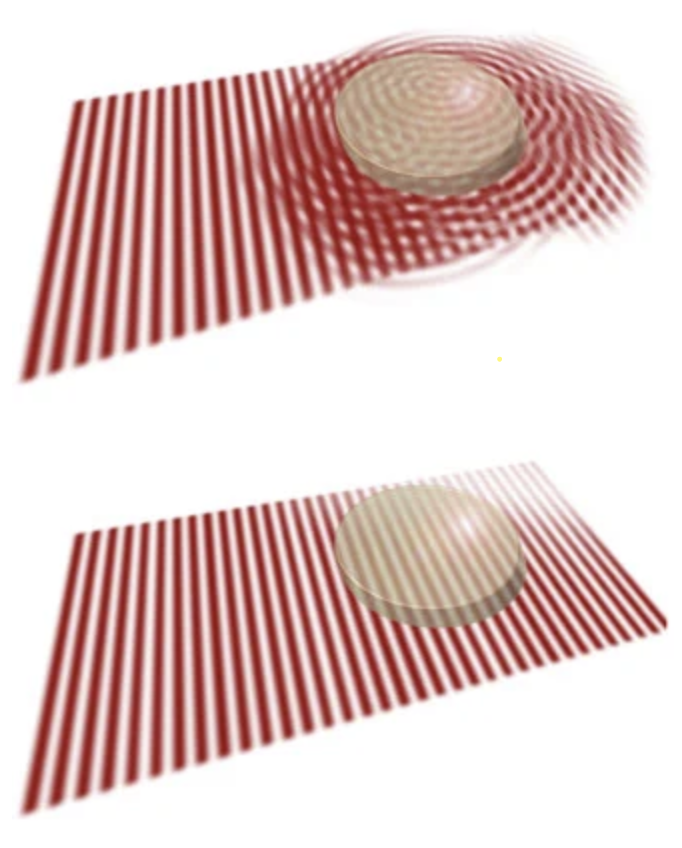
Contrasting Light Behavior: The left panel illustrates a light wave’s uninterrupted journey through a Non-Hermitian material, retaining uniform brightness across its path. The right panel showcases the altered state of a light wave as it navigates through a conventional material, marked by a kaleidoscope of light intensities due to interference. Image courtesy of TU Wien.
In contrast to the distortion typical in traditional materials, Non-Hermitian materials are crafted to maintain a light wave’s uniform intensity by locally amplifying or absorbing light, counteracting scattering and diffraction. This innovation allows light to traverse these materials as smoothly as it would through free space, rendering the materials virtually “invisible” to the light wave, as described by Konstantinos Makris from TU Wien.
Each of these material types marks a significant stride in the field of invisibility, showcasing the diverse approaches and innovative technologies aimed at mastering the art of making objects unseen. These developments open the door to a myriad of applications that were once deemed the realm of fantasy.
5. Manufacturing Invisible Materials
Creating invisible materials involves advanced fabrication processes, from precise nanoscale assembly to sophisticated metamaterial engineering. Techniques like lithography, chemical vapor deposition, and self-assembly are pivotal, each catering to the intricate designs necessary for manipulating light at microscopic levels.
Scaling these materials for commercial use presents significant challenges. The complexity of producing materials with precise nanostructures or negative refractive indices demands high precision and control, often leading to high production costs and technical hurdles. Additionally, durability and adaptability for real-world applications remain critical considerations, necessitating further innovation in material science and engineering.
Looking ahead, the future of manufacturing invisible materials lies in breakthroughs in scalable production techniques and the integration of smart, adaptable materials. Advances in 3D printing, nanofabrication, and material synthesis are set to revolutionize the field, making invisibility technologies more accessible and applicable across industries, from stealth technology to healthcare.
6. Applications of Invisible Materials
Invisible materials are transcending theoretical boundaries, finding their place in diverse sectors:
Stealth Technology: In military and defense, these materials are pivotal in developing stealth technology, reducing radar and infrared signatures of vehicles and equipment. Current implementations include radar-absorbing coatings and meta-surfaces that bend electromagnetic waves. The future may see complete invisibility cloaks, enhancing the efficacy of covert operations.
Medical Field: In healthcare, invisible materials revolutionize imaging and treatment. Metamaterials, for example, are used in enhancing MRI imaging quality, providing clearer, more detailed scans. Looking forward, they hold potential in non-invasive surgeries and targeted therapy, promising minimal patient discomfort and quicker recovery times.
Consumer Electronics: The realm of consumer electronics benefits from invisible materials in creating advanced displays and optical devices. Transparent, flexible displays are just the beginning, with ongoing research aiming to integrate invisibility cloaks into everyday gadgets, potentially revolutionizing user interfaces and device interactivity.
Sustainable Energy: In sustainable energy, these materials boost solar cell efficiency and light management in photovoltaic devices, enabling higher energy conversion rates. Future advancements may lead to entirely transparent solar panels, seamlessly integrating into windows and buildings for urban energy generation without aesthetic disruption.
Each of these applications not only showcases the current utility of invisible materials but also hints at their vast potential to reshape industries and daily life in profound ways.
7. Ethical and Social Considerations
The advent of invisibility technology brings with it a complex web of ethical and social implications that necessitate careful consideration:
Ethical Implications: The potential of invisibility to alter the very fabric of societal norms and personal interactions raises profound ethical questions. The ability to become unseen could be leveraged for espionage or to bypass security measures, challenging existing legal frameworks and ethical boundaries. As such, the development and deployment of these technologies must be guided by stringent ethical standards to prevent misuse.
Privacy Concerns: Privacy emerges as a paramount concern, with invisibility technology posing risks to personal and national security. The prospect of undetectable surveillance devices, for instance, underscores the need for robust privacy protections and regulations to safeguard individuals and institutions from invasive observations and data breaches.
Regulatory and Safety Considerations: Regulating the development and use of invisible materials is crucial to ensure they are safe, effective, and do not pose unforeseen risks to people or the environment. This involves rigorous testing, certification processes, and the establishment of international standards to govern their application across various domains. Moreover, safety protocols must be developed to address potential hazards, from environmental impact to health risks, ensuring that the benefits of invisibility technology do not come at the cost of public well-being.
Navigating these considerations requires a multidisciplinary approach, involving ethicists, lawmakers, scientists, and the public, to create a framework that balances innovation with ethical responsibility, privacy, and safety.
8. Future of Invisible Materials
The future of invisible materials is a dynamic field marked by rapid advancements and interdisciplinary efforts. Cutting-edge research in quantum computing, nanotechnology, and photonics is paving the way for enhanced cloaking devices capable of handling a wider spectrum of electromagnetic waves, inching closer to the once speculative goal of perfect invisibility. The fusion of physics, materials science, engineering, and computer science is crucial, as collaborative innovation helps overcome existing barriers and opens up new possibilities.
As we look ahead, invisible materials promise to redefine the interplay between technology and daily life, offering a more integrated approach where technology blends seamlessly into our surroundings. This evolution is not just about the technological feats but also about envisaging a future where these innovations enrich human experiences, promote environmental sustainability, and address ethical considerations. The potential for invisible materials to transform areas ranging from personal privacy to urban design is immense, signaling a shift towards a world where the boundaries between the visible and the invisible are fluid, and the applications of such materials are woven into the fabric of society.
9. Conclusion
This article has taken us on a trip into the world of invisible materials, showing us the science behind making things invisible, the special materials used for this purpose, and the big changes they’re starting to bring to different areas like the military, healthcare, and green energy. The idea of making things invisible, which once seemed like something out of a fairy tale, is now becoming real thanks to a lot of smart people working in this exciting field.
Continuing to explore and improve these invisible materials is really important. Each new discovery could open up new possibilities we haven’t even thought of yet, from gadgets that work better and look cooler to new ways of treating diseases that are less scary for patients. This is an open call to everyone, whether you’re a scientist in a lab coat or just someone who’s curious about the world, to join in on this adventure into the invisible. The journey is far from over, and there’s so much more out there waiting to be uncovered. With every step forward, we’re not just learning more about invisibility; we’re also unlocking new potential to make our world a better place.
10. References and Further Reading
In crafting this article, insights were drawn from a blend of scientific research, expert analyses, and reputable publications in the field of materials science and optics. To further your understanding and exploration of invisible materials, consider delving into the following resources:
References from Academic Journals:
- Cai, W., Chettiar, U. K., Kildishev, A. V., & Shalaev, V. M. (2007). Optical cloaking with metamaterials. Nature Photonics, 1(4), 224–227. https://doi.org/10.1038/nphoton.2007.28
- He, S., Schog, S., Chen, Y., Ji, Y., Panitz, S., Richtering, W., & Göstl, R. (2023). Photoinduced Mechanical Cloaking of Diarylethene-Crosslinked Microgels. Advanced Materials. https://doi.org/10.1002/adma.202305845
- Alù, A., & Engheta, N. (2009). Cloaking a Sensor. Physical Review Letters, 102(23), 233901. https://doi.org/10.1103/PhysRevLett.102.233901
Books:
- “Invisible: The Dangerous Allure of the Unseen” by Philip Ball – A compelling read that examines invisibility from historical, scientific, and philosophical perspectives.
- “Metamaterials: Theory, Design, and Applications” by Tie Jun Cui, David R. Smith, and Ruopeng Liu – Offers a deep dive into the science and engineering of metamaterials, suitable for both novices and experts. https://link.springer.com/book/10.1007/978-1-4419-0573-4
Other Useful Sources:
- Using Optical Cloaking to Hide Objects in Plain Sight – The FindLight blog explores how metamaterials are used in optical cloaking, expanding their application beyond lens design to innovative cloaking technologies
- The Optical Society (optics.org) – Provides updates on the latest in optical research and technology.
- Photonics.com – A comprehensive portal for news, trends, and educational resources in the field of photonics and light-based technologies.
Each of these references offers a unique lens through which to view the evolving landscape of invisible materials, catering to a wide range of interests from foundational concepts to cutting-edge research. Whether you’re an aspiring scientist, an established engineer, or simply captivated by the possibilities of invisibility, these resources will enrich your journey into this intriguing field.