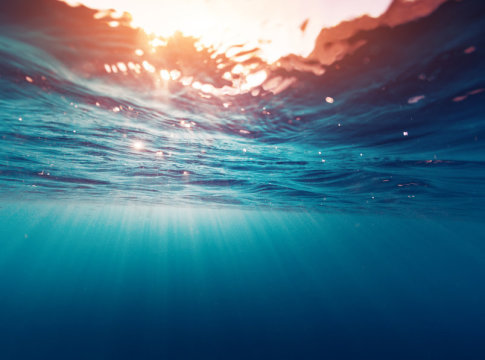
Figure 1 – A research team from the University of Illinois developed an underwater GPS by using light polarization to pinpoint one’s location while underwater. Picture courtesy of ScienceDaily.
Researchers from the University of Illinois recently developed a new method of navigating while underwater by taking advantage of light polarization. This underwater GPS has the ability to accurately pinpoint an individual’s position on Earth within just a few dozen kilometers. In addition to this technology’s ability to improve ocean navigation, it also has several impressive applications in modern medicine, such as tumor detection
This exciting advancement was inspired by the physiology of a mantis shrimp’s eye. Unlike human eyes, mantis shrimp’s eyes are very sensitive to the polarization of light and can even see ultraviolet light. Many other common organisms also have this ability to sense the polarization of light, and can use this knowledge to their advantage. For example, birds that travel long distances during migration will take advantage of light’s polarization to “calibrate their magnetic compasses,” and help them to reach their destination. Given how well this works for birds, these researchers believed that it should also be possible for humans to navigate using light.
Light Polarization
Simply put, the polarization of a light wave is the direction in which its electric field oscillates. Most commonly, light exists in an unpolarized form, where the electric field oscillates in many different, or random, directions.
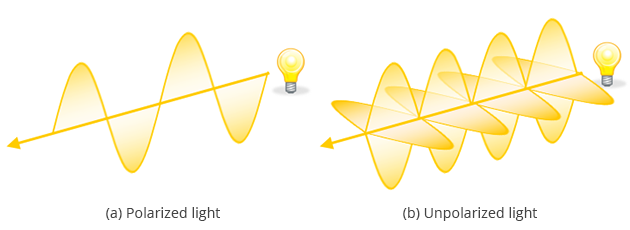
Figure 2- Polarization refers to the direction in which light’s electric field oscillates. If there are multiple directions in which the field oscillates , then the light is said to be unpolarized. Picture from Bigshot Camera.
A number of different factors can cause unpolarized light to either become polarized or can affect the direction of light’s existing polarization. Artificially, polarizers are used in labs and in everyday life to manipulate light’s polarization. Many scientists use polarizing filters in order to create a uniform polarization for laboratory experiments. Others use very similar polarizers in their sunglasses to reduce glare and brightness from the sun’s rays so they can see more easily outdoors.
Interactions with water can also affect the polarization of light. This effect is due primarily to scattering and refraction as the light enters the new medium. As shown in the figure below, when light travels from the air into the water, the light rays bend instead of passing directly through from one medium to another. This phenomenon, called refraction, results in deceiving images, because to those outside the water things often appear to be where they are not.
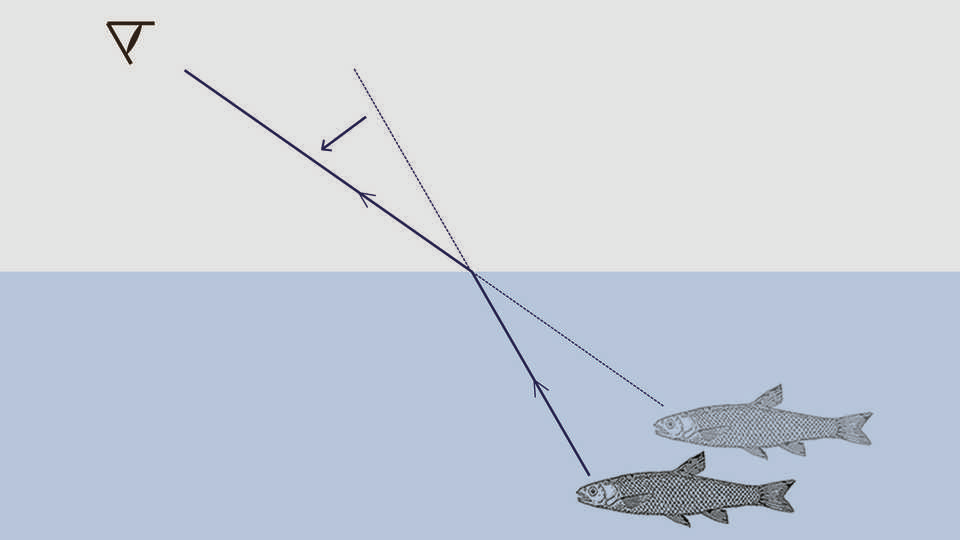
Figure 3 – As light rays transition from air into a new medium (water),
the light rays bend in a phenomenon called refraction. As a result, the fish appears to be further away and nearer to the surface(gray) than it is in reality (black).
Picture from the California Academy of Sciences.
When these light rays enter the water, they also become polarized according to the angle at which the incident, or original, light ray entered the water. Some of the light that enters the water will eventually be reflected at the surface and directed back toward the seafloor. This scattering also serves to further polarize the light. Because that the angle of light polarization depends on the angle of the incident ray, each light ray will have a slightly different polarization that is related to its latitude and longitude. As such, light polarization could conceivably be used as a type of independent coordinate system.
Creating the GPS
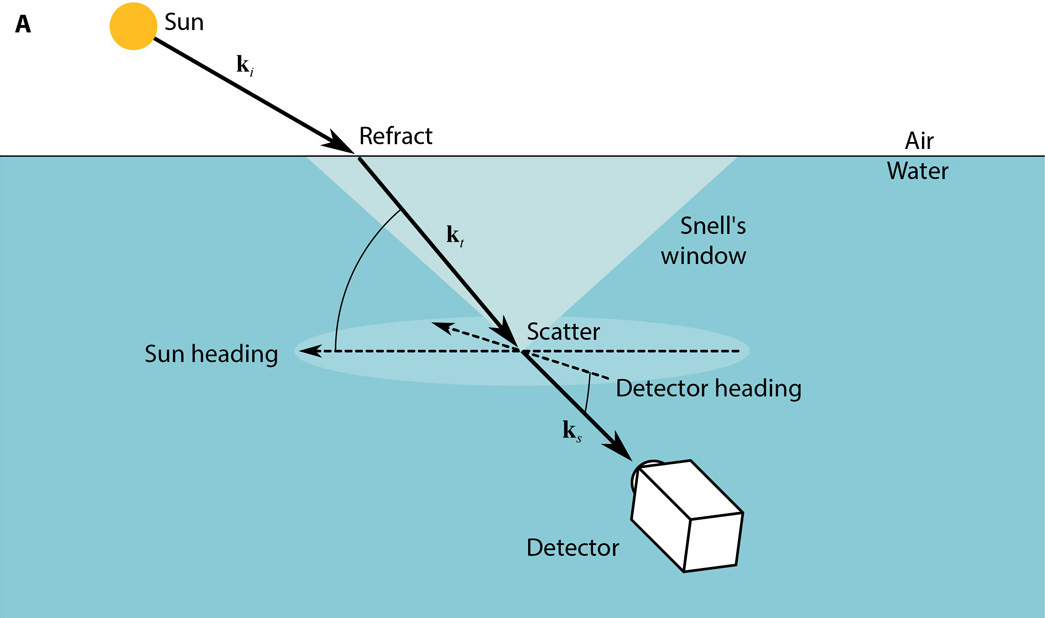
Figure 4 – Polarization video cameras were used to analyze the polarization
of incoming light underwater to predict the detector’s geographic location.
Picture from Powell et al. “Biosinspired Polarization Vision Enables Underwater Geolocalization.”
DOI: 10.1126/sciadv.aao6841
While discovering the connection between light polarization and man-made coordinate systems is significant in and of itself, implementing this GPS system was certainly not without its challenges. For one, the position of the sun relative to one’s position on Earth is continuously changing. Every day the Earth not only completes a full rotation, but also processes about its own central axis. Ever-changing weather conditions also have the potential to affect underwater light’s polarization angle and further complicate matters. Fortunately, the majority of these complications are purely mathematical in nature, and were resolved through computing power along with calibration and meticulous measurements.
During the course of the experiment, the researchers also discovered several key sources of error. One of the largest of sources they discovered was that the angle of the sun above the horizon. They found that when the sun was low in the sky (≤ 40˚ above the horizon), the polarization of light entering the water depended significantly on other factors. Therefore, by only taking measurements when the sun was high in the sky (≥ 40˚ above the horizon), the device’s estimates of position improved dramatically. Interestingly, these researchers also realized that there was a consistent source of “error” between their calculated and actual measurements of position, which they predicted were the result of other factors affecting light’s polarization that their model had failed to capture. Subtracting away this additional error also substantially improved the model’s predictive power.
After accounting for these sources of error, the accuracy of the GPS’s location estimates improved dramatically, as shown in the figure below. Locations where data was collected are denoted by a blue cross, and the center of the corresponding location predictions are indicated by a red x. The dashed lines that surround this red x correspond to 1, 2, and 3 standard deviations away from this central point. Picture (C) includes all of the corrective factors discussed, and is able to make an impressive prediction about the scientists’ location.
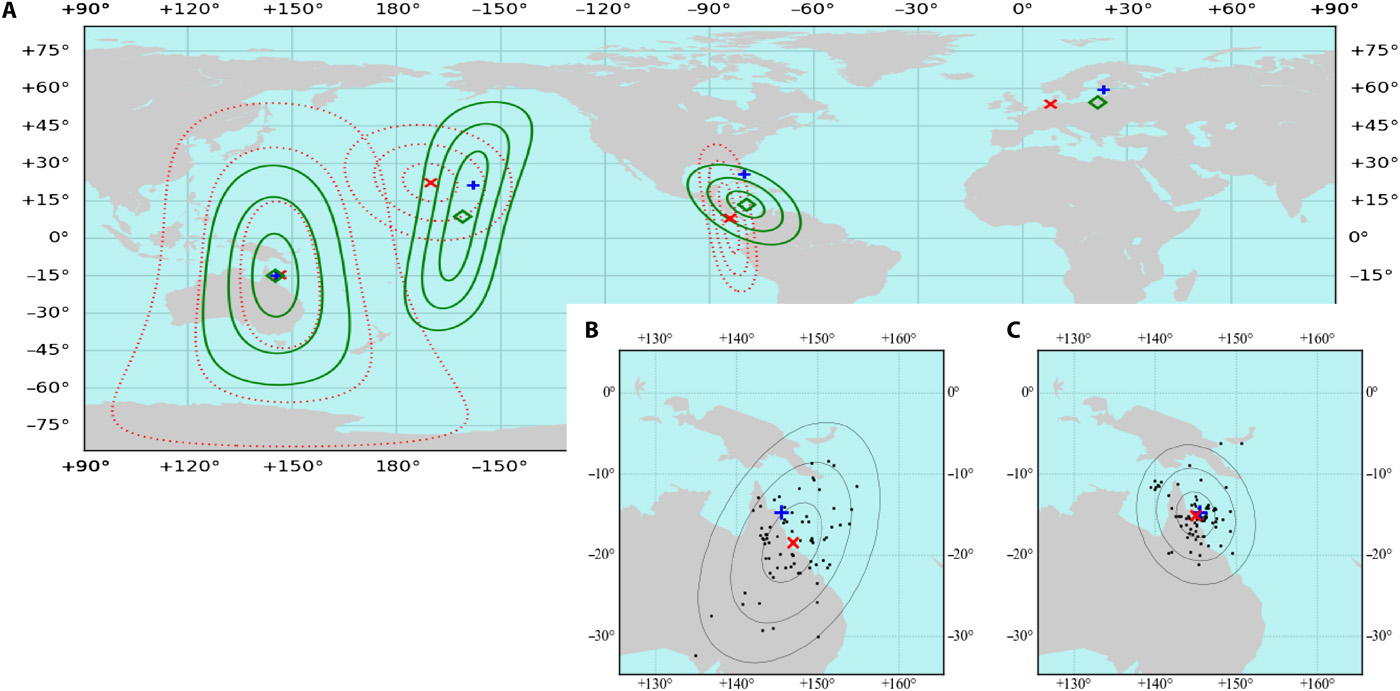
Figure 5- A visual representation of the location estimate. Picture (A) shows the estimates where no error corrections were made. Picture (B) includes only polarization data where the sun was at or above 40˚ above the horizon. Picture (C)’s prediction includes the same correction as (B) and also accounts for the calculated background errors/interactions.
Picture from Powell et al. “Biosinspired Polarization Vision Enables Underwater Geolocalization.”
DOI: 10.1126/sciadv.aao6841
Click here to read more about the scientists’ groundbreaking research.