In the precise and demanding world of optical engineering, the ability to accurately measure and control light is fundamental. Wavefront sensors, the backbone of optical diagnostics, play an indispensable role in achieving this precision. This introduction serves as a deep dive into the technology of wavefront sensors, tailored for engineers who seek a thorough understanding of these essential instruments.
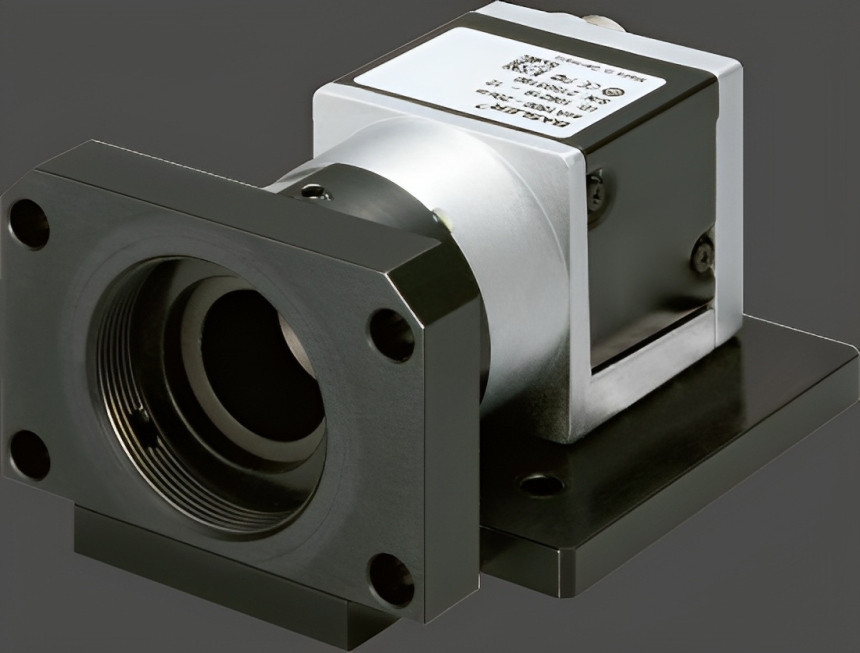
Precision Personified: The Wavefront Sensor in Focus. Photo Courtesy of Pulstec Industrial Co. Ltd.
Wavefront sensors are at the forefront of optical measurement, providing critical data on light wave distortions as they travel through various media and optical systems. This data is crucial for diagnosing and correcting aberrations in a wide range of optical applications – from refining the accuracy of telescopes and enhancing the quality of laser systems to pushing the boundaries of imaging techniques in microscopy.
Understanding the operational principles of wavefront sensors is key to leveraging their full potential. These devices, utilizing methodologies such as Shack-Hartmann, curvature, and shearing interferometry, offer nuanced insights into the phase and intensity distributions of light waves. Each method has its unique advantages, and selection often depends on the specific requirements of the application, such as sensitivity, dynamic range, and spatial resolution.
The engineering applications of wavefront sensors are vast and varied. For astronomical observations, they are indispensable for adaptive optics systems, compensating for atmospheric turbulence to capture clearer images of celestial bodies. In the medical field, particularly in ophthalmology, wavefront sensors enable precise measurements of the eye, leading to better diagnostic procedures and vision correction methods. In laser-based manufacturing and material processing, they ensure the accuracy and quality of laser beams, enhancing the efficiency and precision of these processes.
This technical guide on Wavefront Sensors is brought to you by Gentec-EO - specializing in laser beam and THz source measurement and analysis
What We Cover Here
This introduction lays the groundwork for a comprehensive exploration of wavefront sensor technology, aiming to enrich the knowledge and skills of engineers in this dynamic and critical domain of science. Here is what we cover in this guide:
- Fundamentals of Wavefront Sensing
- Types of Wavefront Sensors
- Technical Details and Comparative Analysis
- Data Processing and Software in Wavefront Sensing
- Applications of Wavefront Sensors
- Advancements and Future Trends in Wavefront Sensing
- Case Studies and Real-World Applications
- Conclusion
- References and Further Reading
1. Fundamentals of Wavefront Sensing
1.1. Basic Principles and Concepts
Wavefront sensing is a critical process in optical engineering, where the primary objective is to measure the shape of an optical wavefront with precision. At its core, a wavefront sensor doesn’t measure the intensity of light, as do traditional imaging devices, but rather the phase and spatial distribution of the light wave, which is essential to understanding the optical quality and aberrations present in an optical system.
The fundamental principle behind wavefront sensing lies in its ability to detect and quantify deviations from an ideal wavefront, typically a plane or spherical wave. These deviations are caused by imperfections in optical components, atmospheric turbulence, or other factors affecting the light’s path. By measuring these deviations, wavefront sensors provide invaluable data for correcting optical systems, ensuring optimal performance.
1.2. Understanding Wavefronts and Their Role in Optics
A wavefront can be thought of as an imaginary surface over which the light wave has a constant phase. In ideal conditions, wavefronts emitted from a point source are spherical, and those from a distant source are planar. However, in practical scenarios, wavefronts often experience distortions as they pass through various media or optical systems.
The role of wavefronts in optics is fundamental. They determine how light converges or diverges, affecting everything from the focus of a camera lens to the resolution of a telescope. Understanding the shape and behavior of these wavefronts is crucial for designing and optimizing optical systems, be it in high-precision telescopes, laser systems, or advanced imaging technologies.
1.3. Common Types of Aberrations Detected by Wavefront Sensors
Wavefront sensors are adept at detecting a wide range of aberrations, which are deviations from the ideal wavefront shape. Common types of aberrations include:
- Spherical Aberration: Occurs when light rays striking the lens or mirror near its edge are not focused at the same point as those hitting near the center.
- Coma: This aberration causes points off the optical axis to appear comet-like, with tails pointing away from the center.
- Astigmatism: Astigmatism in optics results in vertical and horizontal lines being focused at different distances, leading to a blurred image.
- Field Curvature: This causes the image plane to become curved, leading to out-of-focus edges in flat-field imaging systems.
- Chromatic Aberration: It’s a dispersion effect where different wavelengths of light are focused at slightly different points.
Wavefront sensors play a pivotal role in detecting and quantifying these aberrations, allowing optical engineers to make necessary corrections and enhance the performance of optical systems.
2. Types of Wavefront Sensors
Wavefront sensors are instrumental in the field of optics, enabling the precise measurement and analysis of light wavefronts. These sensors come in various forms, each with unique operating principles and suited to specific applications. The choice of a wavefront sensor is critical and depends on factors such as the required resolution, sensitivity, application domain, and environmental conditions. In this section, we will explore the most prominent types of wavefront sensors, delving into their mechanisms, applications, and the advantages and limitations inherent to each. This overview aims to provide a clear understanding of the diverse technologies in wavefront sensing, equipping engineers and students with the knowledge to select and utilize the appropriate sensor for their specific needs.
2.1. Shack-Hartmann Wavefront Sensor
Operating Principles: The Shack-Hartmann sensor consists of an array of lenslets, each focusing light onto a photodetector array. By analyzing the deviations of the focal spots from their expected positions, the wavefront’s shape can be deduced. This method translates the wavefront’s phase information into an intensity pattern, which can be easily measured.
Typical Applications and Use-Cases: This sensor is widely used in astronomy for adaptive optics systems, in ophthalmology for eye examination, and in laser beam shaping. Its ability to accurately measure aberrations makes it invaluable in improving imaging quality and correcting optical systems.
Advantages and Limitations: The primary advantage of the Shack-Hartmann sensor is its robustness and simplicity, making it suitable for a variety of applications. However, its resolution is limited by the number of lenslets, and it may struggle with highly aberrated wavefronts.
2.2. Curvature Sensor
Operational Description: Curvature sensors measure the wavefront’s curvature at various points. This is typically done by focusing the wavefront on a detector at multiple distances and then calculating the curvature based on the intensity variations.
Key Applications: These sensors are useful in situations where space is limited, such as in intraocular lens testing. They are also used in some adaptive optics systems.
Pros and Cons: The curvature sensor is compact and can provide high-speed measurements, which is beneficial in dynamic environments. However, it is generally less sensitive than other types and can be more complex to interpret.
2.3. Shearing Interferometer Wavefront Sensors
Mechanism of Operation: A shearing interferometer works by splitting a wavefront into two or more parts that are later recombined. The interference pattern produced by this recombination is analyzed to determine the wavefront’s characteristics. This method is sensitive to phase shifts, providing detailed information about the wavefront.
Application Domains: It finds use in high-resolution microscopy, optical testing, and laser diagnostics. Its high sensitivity makes it suitable for detecting subtle aberrations in complex optical systems.
Strengths and Weaknesses: Shearing interferometers are highly sensitive and can measure small phase changes accurately. However, they can be complex to set up and interpret, and are typically more sensitive to environmental disturbances.
2.4. Other Notable Types
- Phase-Shifting Interferometer: Utilizes phase shifts in light waves to measure wavefront distortions. Ideal for high-precision measurements in controlled environments.
- Foucault Knife-Edge Test: A simple, yet effective method primarily used in amateur telescope making for evaluating the quality of optical surfaces.
- Hartmann Test: An older method where a plate with holes is used to sample the wavefront, primarily used for testing large, astronomical optics.
Each of these sensors has its unique strengths and is chosen based on the specific requirements of the application, such as resolution, sensitivity, and environmental factors.
3. Technical Details and Comparative Analysis
In this section, we delve into the intricate technical details of wavefront sensors and offer a comparative analysis of their performance characteristics. Understanding these technical nuances is crucial for engineers and students, as it helps in selecting the right sensor for specific applications and in appreciating the engineering challenges involved in wavefront sensing.
3.1. Operational Principles and Technical Specifications
Shack-Hartmann Wavefront Sensor: The technical prowess of this sensor lies in its array of lenslets, each creating a focal spot. The position of these spots relative to a reference point indicates the wavefront’s local slope. Key specifications include lenslet array size, spot detection accuracy, and dynamic range.
Curvature Sensor: This sensor’s capability hinges on its differential measurement approach, taking readings at different zonal depths. Technical considerations include the radius of curvature of the reference wavefront, sensitivity to intensity fluctuations, and response speed.
Shearing Interferometer: The shearing interferometer’s strength lies in its ability to compare different segments of the wavefront, allowing for detailed phase analysis. Important technical details include shear amount, phase sensitivity, and interference pattern resolution.
3.2. Comparative Analysis Based on Key Performance Metrics
Precision and Sensitivity: Shack-Hartmann sensors generally offer robust precision suitable for a range of applications, but may lack the high sensitivity of shearing interferometers, which excel in detecting minute phase shifts.
Resolution and Dynamic Range: While Shack-Hartmann sensors have a resolution limited by the lenslet array, curvature sensors and shearing interferometers can offer higher resolution measurements. The dynamic range, a critical factor for applications like astronomical observations, varies significantly among these types, with Shack-Hartmann sensors typically providing a broader range.
Environmental Robustness and Practical Usability: The Shack-Hartmann sensor is often lauded for its simplicity and robustness in diverse environments. In contrast, shearing interferometers, while highly sensitive, can be more susceptible to environmental factors like vibration and temperature changes.
3.3. Discussion on Hardware Components
Lenslet Arrays in Shack-Hartmann Sensors: The design and quality of lenslet arrays are pivotal, with factors like lenslet size and array configuration impacting the sensor’s performance.
Photodetectors and CCD Cameras: High-quality photodetectors and CCD cameras are essential across all sensor types for accurate wavefront measurement. The resolution, sensitivity, and noise characteristics of these components directly affect the quality of the wavefront measurement.
Optical and Mechanical Design: The overall design, including optical alignment, stability, and mechanical robustness, plays a significant role in the performance and longevity of these sensors.
4. Data Processing and Software in Wavefront Sensing
In wavefront sensing, the acquisition of raw data is just the beginning. The true essence of wavefront analysis lies in the processing and interpretation of this data, which is achieved through sophisticated algorithms and software tools. This section will explore the critical aspects of data processing in wavefront sensing, highlighting the algorithms used, the role of software tools, and addressing the challenges faced in processing wavefront data.
4.1. Algorithms Used for Wavefront Reconstruction
Zernike Polynomial Decomposition: Widely used for its ability to represent wavefront distortions in a series of orthogonal polynomials, making it highly effective for analyzing and correcting aberrations.
Fourier Transform Methods: Utilized for their efficiency in reconstructing phase information, especially in systems like shearing interferometers.
Least Squares and Iterative Methods: Often employed in Shack-Hartmann wavefront sensors to calculate the wavefront shape from spot displacements, balancing accuracy and computational load.
4.2. Software Tools Commonly Employed in Wavefront Analysis
Wavefront Analysis Software: Specialized software packages are available for different types of wavefront sensors, each designed to maximize the potential of the respective sensing technology. These tools often feature user-friendly interfaces, real-time processing capabilities, and advanced data visualization options.
Custom Algorithm Development: For specific or advanced applications, engineers and researchers may develop custom algorithms tailored to their unique requirements, leveraging programming languages like MATLAB or Python.
Integration with Optical Design Software: Wavefront data is often used in conjunction with optical design software to simulate, analyze, and optimize optical systems, bridging the gap between theoretical design and real-world performance.
4.3. Challenges in Data Processing and Potential Solutions
Handling Large Data Volumes: Modern wavefront sensors can generate vast amounts of data, posing a challenge in terms of storage and processing speed. Solutions include optimized algorithms, efficient data management practices, and the use of high-performance computing resources.
Noise Reduction and Error Analysis: Wavefront data can be affected by various sources of noise and errors. Advanced filtering techniques, error correction algorithms, and rigorous calibration procedures are employed to enhance data fidelity.
Adapting to Dynamic Conditions: In applications such as adaptive optics, wavefront sensors must process data in real-time and adapt to rapidly changing conditions. This requires robust algorithms and high-speed processing capabilities, often achieved through dedicated hardware or parallel processing techniques.
5. Applications of Wavefront Sensors
Wavefront sensors have a broad range of applications, revolutionizing fields from astronomy to medical science. Their ability to precisely measure and correct optical aberrations makes them indispensable tools in numerous areas. Let’s explore some of these key applications.
Astronomy and Adaptive Optics: In astronomy, wavefront sensors are crucial. They correct for atmospheric distortion, allowing telescopes to capture clearer images of celestial bodies. Adaptive optics systems, equipped with these sensors, dynamically adjust mirrors. This corrects for the blurring effect of the Earth’s atmosphere.
Vision Science and Ophthalmology: In ophthalmology, wavefront sensors have transformed eye care. They provide detailed maps of eye aberrations, enhancing diagnostic procedures. This leads to more accurate vision correction methods. It’s a leap forward from traditional eye examinations.
Laser Systems and Optical Testing: In the field of laser systems, wavefront sensors ensure beam quality and alignment. They are essential for applications ranging from material processing to laser eye surgery. In optical testing, these sensors verify the performance of lenses and mirrors. This ensures they meet strict quality standards.
Emerging Applications in New Fields: Emerging applications are continually surfacing. For instance, wavefront sensors now aid in high-resolution microscopy and telecommunications. Their ability to analyze light properties expands the possibilities in these fields. They promise to drive future innovations.
6. Advancements and Future Trends in Wavefront Sensing
Wavefront sensing technology is rapidly evolving, bringing new advancements and setting trends that shape the future of optical engineering. This forward momentum not only enhances current applications but also opens doors to unexplored possibilities. Let’s delve into these recent developments and anticipated trends.
Recent Technological Advancements: Recent years have seen significant breakthroughs in wavefront sensor technology. Enhanced sensitivity and resolution are notable improvements. Miniaturization has also been a key focus, leading to more compact and portable sensors. Integration with artificial intelligence (AI) and machine learning algorithms is another leap forward. It offers improved data analysis and real-time adaptive corrections.
Anticipated Future Trends: Looking ahead, the trend towards integration and multi-functional sensors is evident. These sensors will combine various measurement techniques for comprehensive analysis. The use of advanced materials and nanotechnology promises even smaller, more efficient sensors. There’s also a growing emphasis on real-time processing capabilities, crucial for dynamic applications like autonomous vehicles and robotic surgery.
Emerging Challenges and Opportunities: As technology advances, new challenges emerge. Ensuring data accuracy and reliability in increasingly complex systems is a major concern. Addressing these challenges requires innovative approaches and continuous research. However, these challenges also present opportunities. They drive the development of more sophisticated wavefront sensing solutions, further expanding the technology’s potential.
7. Real-World Applications of Wavefront Sensors
The practical impact of wavefront sensors becomes most apparent through case studies and real-world applications. These examples not only illustrate the technology’s versatility but also provide valuable insights and best practices. Let’s examine a few notable instances where wavefront sensors have made a significant difference.
Improving Astronomical Observations: One notable case study involves the use of adaptive optics in large telescopes. Wavefront sensors here have drastically improved the resolution of astronomical images. By compensating for atmospheric turbulence, these sensors have allowed clearer views of distant galaxies and celestial phenomena, leading to groundbreaking discoveries in astronomy.
Advancements in Ophthalmology: In the field of eye care, wavefront sensors have revolutionized vision correction procedures. A key example is their use in customizing laser eye surgery, where they map individual eye aberrations. This ensures highly personalized and accurate corrections, significantly enhancing patient outcomes.
Enhancing Laser System Efficiency: In industrial applications, wavefront sensors play a crucial role in laser-based manufacturing processes. For instance, their use in ensuring the precision and quality of laser cutting systems has led to improved efficiency and product quality, showcasing their importance in high-precision manufacturing environments.
Breakthroughs in Microscopy: Another application area is in high-resolution microscopy. Here, wavefront sensors have enabled more detailed and accurate imaging of biological specimens. This has been instrumental in advancing research in fields like cellular biology and neuroscience.
8. Conclusion: The Pivotal Role of Wavefront Sensors in Optical Engineering
As we conclude our exploration of wavefront sensors, it’s clear that these tools are more than just intricate pieces of optical technology; they are fundamental enablers in the advancement of optical engineering and related fields. This guide has delved into the complex nature of wavefront sensors, revealing their multifaceted applications and the technical ingenuity behind them.
Wavefront sensors stand at the intersection of theory and practice, transforming abstract optical concepts into tangible measurements and corrections. From the basic principles of wavefront distortion to the advanced operational intricacies of Shack-Hartmann, Curvature, and Shearing Interferometer sensors, we have seen how each type serves a unique purpose. Their diverse applications, ranging from enhancing astronomical observations to driving innovations in medical diagnostics, underscore their versatility.
The technical evolution of these sensors, marked by advancements in resolution, sensitivity, and real-time processing, mirrors the rapid progress in optical engineering. The future of wavefront sensing, poised for integration with AI and machine learning, promises even more refined and adaptable optical solutions. However, this journey is not without its challenges. The need for accuracy and reliability in increasingly complex systems continues to spur innovation and research.
In summary, wavefront sensors are indispensable in the quest for optical precision. They are key to understanding and manipulating light, and their role in shaping future optical technologies cannot be overstated. As we look forward to new developments in this field, the importance of continuous learning and adaptation becomes ever more apparent. Wavefront sensors, in their varied forms and functions, will undoubtedly continue to be at the forefront of optical advancements, paving the way for new discoveries and applications.
If you are enjoying this article, you might also like our Guide on 3D Cameras.
9. References and Further Reading
To deepen your understanding of wavefront sensors and stay abreast of the latest developments in this field, the following curated list of references and resources is invaluable. These materials range from foundational academic papers to advanced textbooks and are essential for anyone looking to expand their knowledge in optical engineering and wavefront sensing.
9.1. Academic Papers and Journals
- “Wavefront detection method of a single-sensor based adaptive optics system,” by Chongchong Wan et al. A seminal paper discussing the principles of adaptive optics and the role of wavefront sensors.
- “Advanced Optical Wavefront Technologies to Improve Patient Quality of Vision and Meet Clinical Requests” featured in Polymers. This paper offers insights into the application of wavefront sensing in vision science.
9.2. Textbooks and Handbooks on Wavefront Sensors
- “Principles of Adaptive Optics” by Robert K. Tyson. A comprehensive textbook covering the basics and advanced concepts in adaptive optics, including wavefront sensing techniques.
- “Handbook of Optical Engineering” edited by Daniel Malacara. This handbook includes a section dedicated to wavefront sensing technologies, offering a detailed overview of various sensor types and their applications.
9.3. Online Resources and Tutorials
- Online Course on Optical Engineering: Many universities and educational platforms offer courses that cover wavefront sensing and its applications in optical engineering.
- Webinars and Lectures: Professional societies like SPIE and OSA regularly host webinars and lectures by experts in the field, discussing the latest trends and research in wavefront sensing.
9.4. Video Tutorials on Wavefront Sensors
The following is a 1 min tutorial on Shack-Hartmann Sensors explains the reconstruction of the wavefront and characterization of aberrations by measuring the local slope of an incoming wavefront and analyzing the displacement of spots generated by a lenslet array:
The following is is a 18 min tutorial on modern wavefront sensing technology. Introduction to wavefront sensing technology. Description of how to use the Thorlabs wavefront sensor software and the Phasics wavefront sensor software.
9.5. FindLight Marketplace
If you are in the market for wavefront sensors, you should check out our dedicated category page for wavefront sensors featuring a variety of sensors from Shack Hartmann, to Lateral Shearing, to Phase-Shifting Interferometer.