Introduction
Interferometry stands as a pivotal technique in modern measurement and metrology, offering unparalleled precision in the examination of surfaces and optical phenomena. At the heart of this approach lies the ability to extract intricate information by observing the interference patterns formed when coherent waves interact. Within this landscape, White Light Interferometry (WLI) emerges as a powerful variant that combines the advantages of traditional interferometry and confocal microscopy.
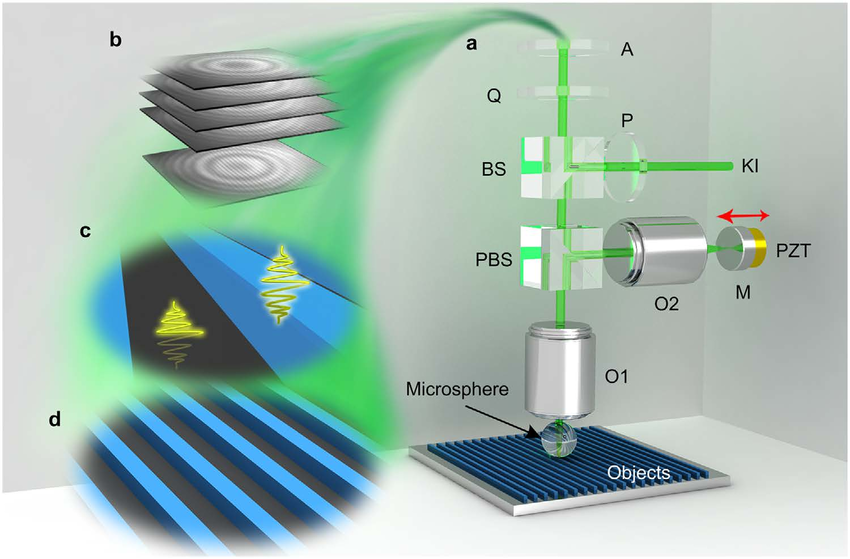
Near-field-assisted-white-light-interferometry. Image Courtesy of Research Gate
Surface topography and roughness play crucial roles in various industries, from microelectronics to precision optics and beyond. Interferometric techniques, by capitalizing on the wave nature of light, provide sub-nanometer precision in measuring these critical features. WLI extends this precision by employing a broadband light source, enabling the capture of a wide range of interference patterns simultaneously. This leads to rapid acquisition of detailed surface profiles with exceptional accuracy.
By using a Michelson interferometer setup and exploiting the interference generated from a spectrum of wavelengths, WLI achieves not only high-resolution imaging but also the ability to measure transparent and reflective surfaces that challenge other methods. This article delves into the principles, setup, applications, and potential future directions of White Light Interferometry, highlighting its vital role in pushing the boundaries of surface metrology and opening avenues for advancements in multiple industries.
Today's post is sponsored by DataRay - leading manufacturer of ISO compliant laser beam profiler
Principles of White Light Interferometry
Interferometry, a cornerstone of precision measurement, exploits the wave nature of light to extract detailed information about surfaces and optical phenomena. White Light Interferometry (WLI) stands as a refined version of this technique, merging the strengths of traditional interferometry and confocal microscopy.
In WLI, a key principle involves the generation of interference patterns by combining light waves of different wavelengths. This is achieved using a Michelson interferometer setup, where a beam splitter directs light to both a reference arm and a test arm. The light waves traverse these paths and recombine, leading to the formation of interference fringes. Importantly, WLI employs a broadband light source, ensuring that a range of wavelengths is utilized. This results in a multitude of interference patterns, which, when analyzed, yield comprehensive surface information.
The optical path difference (OPD), a crucial parameter in interferometry, represents the disparity in the lengths traveled by the light waves in the reference and test arms. By capturing and analyzing the resulting interference patterns—interferograms, pictured above—WLI translates minute OPD variations into three-dimensional surface profiles. This enables high-precision measurements of surface roughness, step heights, and other topographical features.
In summary, the principles of WLI revolve around harnessing interference patterns from a spectrum of wavelengths to derive intricate surface details. This amalgamation of traditional interferometry and modern microscopy techniques enhances the accuracy and versatility of surface metrology, propelling advancements in fields ranging from nanotechnology to manufacturing.
Interference and Optical Path Difference
Central to White Light Interferometry is the phenomenon of interference. This occurs when two or more coherent waves combine to create regions of reinforcement and cancellation. In WLI, interference arises from the interaction of light waves of varying wavelengths, leading to the generation of interference patterns that unveil intricate surface information.
A fundamental concept in interferometry is the optical path difference (OPD), the variance in distance traveled by the light waves in different arms of the interferometer. In the Michelson interferometer setup employed by WLI, a beam splitter splits incident white light into two paths: the reference arm and the test arm. The light waves traverse these paths, encounter the sample in the test arm, and reunite at the beam splitter. Depending on the sample’s characteristics, the optical path traversed by the light in the test arm differs, leading to a varied OPD.
As the recombined waves merge at the detector, they interact, resulting in constructive and destructive interference patterns. The detector captures these patterns in the form of interferograms—visual representations of the variations in intensity caused by interference. These interferograms encode vital surface information, such as elevation changes and roughness.
Deciphering interferograms requires advanced signal processing techniques, including Fourier Transform algorithms. This mathematical operation converts the interference patterns from the time domain to the frequency domain, enabling the extraction of spatial frequency components associated with the surface features. Ultimately, this process unveils the intricate topography of the sample.
To sum up, White Light Interferometry exploits interference patterns generated by the interaction of light waves with varied optical paths. The resulting interferograms, processed through advanced algorithms, serve as the foundation for capturing high-resolution, three-dimensional surface profiles, making WLI an indispensable tool in nanotechnology, material science, and various manufacturing applications.
Michelson Interferometer and WLI Setup
The Michelson interferometer serves as the cornerstone of White Light Interferometry, facilitating the precise measurement of surface topography and roughness. This setup capitalizes on the interference of light waves to extract valuable information about samples.
In the Michelson configuration, incident white light is divided into two paths by a beam splitter. One path—the reference arm—remains static, while the other—the test arm—passes through the sample being analyzed. This dual pathway system introduces a controlled OPD between the two arms. When the light waves recombine at the beam splitter, they generate interference patterns that ultimately reveal the sample’s surface characteristics.
WLI distinguishes itself by utilizing a broadband light source. Unlike traditional single-wavelength interferometry, which produces fringes sensitive to environmental factors, WLI’s broad spectrum allows for the capture of multiple fringes concurrently. This enables rapid and accurate acquisition of detailed surface profiles.
The interference patterns produced are captured by a detector and presented as interferograms. These patterns encode elevation changes, slopes, and other surface features. By applying advanced signal processing techniques, such as Fourier Transform analysis, these interferograms are transformed into high-resolution, three-dimensional surface profiles.
In essence, the Michelson interferometer in White Light Interferometry serves as a platform to leverage the interaction of coherent light waves. This system’s synergy with a broadband light source sets the stage for precise and efficient measurements of surfaces, making WLI indispensable in fields ranging from microelectronics to precision manufacturing.
Broadband Light Source
A defining feature of WLI is its utilization of a broadband light source, a pivotal component that underpins the technique’s precision and versatility. Unlike traditional interferometry methods that rely on monochromatic light, WLI employs a range of wavelengths, enabling comprehensive surface analysis.
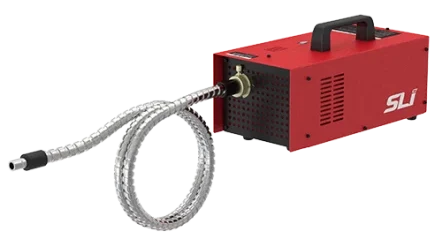
Broadband Light Source at FindLight
The broadband light source generates a spectrum of wavelengths, covering a broad range of colors. When this light is directed into the Michelson interferometer setup, it generates interference patterns containing multiple fringes. Each fringe corresponds to a specific wavelength, collectively forming a complex interferogram.
This diversity of wavelengths has distinct advantages. Traditional interferometers are highly sensitive to environmental factors and require careful isolation, but WLI’s spectrum inherently reduces susceptibility to external disturbances. The multiple fringes obtained from the broadband light source ensure robustness against variations in the test environment.
Furthermore, the wealth of information encoded within the interferogram allows for more accurate analysis. By using advanced signal processing techniques, such as Fourier Transform algorithms, WLI can separate the individual wavelength contributions, translating them into surface profiles with sub-nanometer precision.
Interferogram Analysis
Central to White Light Interferometry is the analysis of interferograms, the complex patterns that encapsulate surface information within WLI measurements (pictured above). These patterns are derived from the interference of light waves and serve as the foundation for capturing detailed surface profiles.
Interferograms are obtained by directing the combined light waves from the Michelson interferometer’s reference and test arms to a detector. These patterns visually represent the variations in intensity resulting from the constructive and destructive interference of light waves. Each fringe in the interferogram corresponds to a specific optical path difference (OPD) between the reference and test arms, which is directly related to the sample’s surface features.
Deciphering the interferogram necessitates advanced signal-processing techniques. One such technique is the Fourier Transform, which converts the interferogram from the time domain to the frequency domain. This transformation disentangles the complex interference patterns into distinct spatial frequency components. Each frequency component corresponds to a specific surface feature, such as elevation changes or roughness.
The process of interferogram analysis enables the extraction of quantitative surface information with remarkable precision. By quantifying the relationships between the OPD and the wavelengths of the light source, the technique translates the interferogram’s fringe patterns into detailed 3D surface profiles.
Advantages of White Light Interferometry
WLI presents a gamut of advantages that position it as a premier technique for high-precision surface metrology, offering unique strengths compared to traditional methods. In this section, we highlight the key advantages that set WLI apart and underscores its significance in diverse industries.
1. Non-Destructive and High-Speed Measurements:
WLI enables non-destructive surface analysis, preserving delicate samples while delivering exceptional accuracy. Unlike contact methods such as stylus profilometry, WLI’s non-contact nature prevents damage, making it suitable for fragile materials and intricate structures. Moreover, its rapid data acquisition speeds streamline quality control and production processes.
2. High-Resolution Imaging:
With WLI, sub-nanometer resolution is achievable, surpassing the capabilities of many other surface metrology techniques. The interferometric nature of WLI allows it to discern features and structures with unparalleled precision, making it indispensable in applications that demand meticulous detail.
3. Versatility in Sample Types:
WLI overcomes challenges posed by transparent and reflective surfaces, which can confound traditional methods. Its ability to analyze a wide range of materials, including semiconductors, metals, ceramics, and biological samples, makes it adaptable to various industries and research domains.
4. Three-Dimensional Profiling:
WLI captures not only 2D profiles but also full 3D surface maps, revealing comprehensive information about surface roughness, step heights, and complex geometries. This dimensional insight is vital in fields such as microelectronics and optics, where intricate structures define performance.
5. Reduced Environmental Sensitivity:
The use of a broadband light source in WLI makes it less susceptible to environmental disturbances compared to traditional interferometers. This robustness enhances measurement reliability, particularly in environments with fluctuating conditions.
All things considered, White Light Interferometry’s blend of non-destructive capabilities, high-resolution imaging, adaptability to various sample types, and comprehensive 3D profiling make it a frontrunner in modern surface metrology. Its ability to swiftly and precisely capture intricate surface characteristics has led to its widespread application in industries like semiconductor manufacturing, aerospace, biotechnology, and beyond, propelling advancements across diverse sectors.
Challenges and Limitations in White Light Interferometry
Although WLI offers remarkable precision and versatility, it is essential to acknowledge the challenges and limitations inherent in the technique. Addressing these factors is crucial for understanding its applicability and potential areas for improvement.
1. Coherence and Fringe Washout:
WLI relies on interference patterns generated by coherent light waves. When the coherence length of the light source is limited, interference fringes can become less distinct or disappear altogether. This can impact measurement accuracy, particularly on samples with complex surface features.
2. Sample Reflectivity and Translucency:
Highly reflective or translucent samples can introduce challenges. Reflective surfaces can cause unwanted reflections and scattering that interfere with the accurate interpretation of interferograms. Translucent materials may absorb and scatter light unevenly, leading to distortion in the interference patterns.
3. Environmental Noise:
While WLI is less sensitive to environmental factors compared to some other interferometric techniques, it is not immune to external vibrations, temperature changes, and air turbulence. These factors can introduce noise and compromise measurement accuracy.
4. Complex Sample Geometries:
Samples with intricate or complex geometries can pose difficulties in interpreting interferograms accurately. Irregular shapes and steep slopes may result in ambiguous fringe patterns that require advanced analysis techniques to unravel.
5. Data Processing Complexity:
Analyzing interferograms and extracting surface information requires sophisticated signal processing techniques like Fourier Transform. Processing large datasets and dealing with noisy data can be computationally intensive and time-consuming.
In summary, White Light Interferometry, while offering remarkable capabilities, faces challenges related to coherence, sample properties, environmental conditions, and data processing. Recognizing and mitigating these limitations is essential for harnessing the full potential of WLI and improving its applicability in various fields.
Applications of White Light Interferometry
Over the years, White Light Interferometry (WLI) has found utility in a myriad of applications ranging from scientific research to industry-specific solutions.
Surface Profiling: One of the primary uses of white light interferometry is in the domain of surface metrology, where it aids in achieving nanometer-scale resolution of surface topographies. Industries, especially semiconductor and data storage, rely on this technique to inspect the uniformity, roughness, and defects on polished surfaces and thin films.
Biomedical Imaging: In the medical world, white light interferometry is a cornerstone of optical coherence tomography (OCT). OCT provides cross-sectional, high-resolution images of biological tissues, enabling the early detection of diseases such as glaucoma and age-related macular degeneration. It’s also used in dermatology to non-invasively visualize skin layers and detect abnormalities.
Material Characterization: By gauging the thickness, refractive index, and dispersion of materials, white light interferometry plays a crucial role in the analysis and quality control of multilayer structures, such as those used in photonic devices.
Microfluidics: In the sphere of lab-on-a-chip technologies and microfluidic applications, white light interferometry offers insights into channel topography, aiding in the design and quality assurance of microfluidic systems.
Environmental Sensing: The sensitivity of interferometric techniques to minute changes in refractive index makes it ideal for environmental monitoring, detecting trace amounts of contaminants in air and water.
Recent Developments and Future Trends
White light interferometry, with its widespread applicability, has been at the forefront of various technological advancements. As researchers uncover its potential, the technique is continually being refined and optimized for better precision and a broader scope.
High-Speed Imaging: The race to achieve faster data acquisition has led to the development of high-speed white light interferometry systems. These systems, utilizing advanced hardware and software integrations, now allow for real-time monitoring and rapid inspections, especially invaluable in industrial quality control and dynamic biological studies.
Extended Depth Imaging: Traditional limitations concerning depth of focus have been mitigated with newer algorithms and system designs. Enhanced depth imaging is particularly beneficial for inspecting complex 3D structures and deep tissue imaging in medical applications.
Integration with AI and Machine Learning: The fusion of white light interferometry with artificial intelligence allows for smarter data interpretation, defect recognition, and automated quality control. AI-driven algorithms can quickly discern patterns and anomalies that might be missed or take longer using traditional methods.
Portable Systems: Miniaturization and improvements in optic technologies have led to the development of compact and portable white light interferometry units. These are game-changers for field studies, on-site industrial inspections, and point-of-care medical diagnostics.
Multimodal Imaging: Combining white light interferometry with other imaging modalities, like Raman spectroscopy or fluorescence microscopy, is a burgeoning trend. This integrative approach offers complementary information, giving a holistic view of the sample under study.
Future Prospects:
The horizon of white light interferometry is brimming with promise. As the demand for non-invasive, high-resolution imaging techniques grows, so will the innovations in this field. Anticipated trends include the development of quantum-enhanced interferometry, offering even higher precision, and advancements in fiber-optic sensors for remote and in-situ monitoring. The continuous push for integration with other technologies will likely open doors to applications yet unimagined.
Conclusion
In the realm of precision metrology, White Light Interferometry (WLI) shines as a cornerstone technique, revolutionizing the way surfaces are characterized. By harnessing the principles of interference and leveraging a broadband light source, WLI offers non-destructive, high-speed, and high-resolution measurements across various materials and industries. Despite challenges related to coherence and sample properties, its advantages are indisputable.
From microelectronics to optics, medical devices, and beyond, WLI’s applications are extensive and far-reaching. Recent developments, such as AI-driven analysis and hybrid approaches, propel it into new realms of efficiency and accuracy. As the future unfolds, WLI’s role in 3D and 4D surface measurements, in-line manufacturing setups, and emerging fields holds immense promise. With its unwavering precision and adaptability, White Light Interferometry continues to be a driving force in advancing technologies, enabling innovation across sectors that demand meticulous surface analysis.