VGEN-SP-NL-25-10 Fiber Laser
Description
Incorporating state-of-the-art laser technology, Spectra-Physics’ VGEN-SP lasers provide top performance in technically demanding LIDAR and range-finding applications. VGEN-SP series of Ytterbium fiber lasers in MOPA configuration offers a constant high peak power over a wide range of pulse repetition rate values for stable high performance. Offering high beam quality, low beam divergence, high peak power and high pulse energy, the VGEN-SP series is competitively priced and offers the full range of specifications to meet a wide spectrum of LIDAR applications. With low weight and small size, the VGEN-SP series is easily deployed. Housed in a robust assembly that meets industrial standards and fitted with a metal armored fiber cable, VGEN-SP lasers deliver a high quality, near diffraction-limited output beam. The laser’s solid construction is maintenance free and reliable, ensuring longlife operation at low operational cost. VGEN-SP lasers are rugged and can stand up to the tough conditions and requirements of airborne LIDAR applications for a robust and stable platform.
VGEN-SP-NL-25-10 Fiber Laser
Specifications |
|
---|---|
Wavelength: | 1064 nm |
Average Output Power: | 10 W |
Pulse Duration: | 1-3ns |
Beam Quality (M^2): | 1.3 |
Max Pulse Repetition Rate: | 700 kHz |
Features
Up to 20 W average output power
Up to 40 kW peak power
35–700 kHz (tunable) repetition rate
Narrow line width down to sub 100 pm
Tested at altitude up to 8,000 m (35.6 kpa) with maximum peak power
High PRF enables higher scanning and data acquisition rates
Comply with MIL-STD-801F Method 500.4
Applications
LIDAR and LADAR
Range finding
Spectroscopy
For pricing, technical or any other questions please contact the supplier
- No registration required
- No markups, no fees
- Direct contact with supplier
-
Ships from:
United States
-
Sold by:
-
On FindLight:
External Vendor
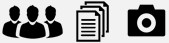
Claim MKS Instruments Page to edit and add data
Frequently Asked Questions
The VGEN-SP laser is used for LIDAR and range-finding applications, as well as spectroscopy.
The VGEN-SP laser has an average output power of up to 20 W and a peak power of up to 40 kW.
The repetition rate of the VGEN-SP laser is tunable between 35-700 kHz.
Yes, the VGEN-SP laser is rugged and can withstand tough conditions and requirements of airborne LIDAR applications.
The VGEN-SP laser has a wavelength of 1064 nm.